- Open access
- Published: 22 October 2021
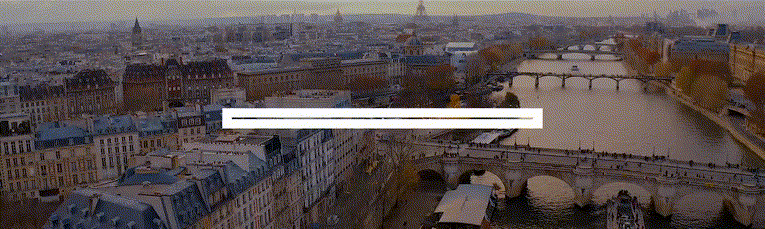
Diaphragmatic excursion is correlated with the improvement in exercise tolerance after pulmonary rehabilitation in patients with chronic obstructive pulmonary disease
- Masashi Shiraishi ORCID: orcid.org/0000-0001-5410-1331 1 , 2 ,
- Yuji Higashimoto 1 ,
- Ryuji Sugiya 1 ,
- Hiroki Mizusawa 1 ,
- Yu Takeda 1 ,
- Shuhei Fujita 1 ,
- Osamu Nishiyama 2 ,
- Shintarou Kudo 3 ,
- Tamotsu Kimura 1 ,
- Yasutaka Chiba 4 ,
- Kanji Fukuda 1 ,
- Yuji Tohda 2 &
- Hisako Matsumoto 2
Respiratory Research volume 22 , Article number: 271 ( 2021 ) Cite this article
4825 Accesses
9 Citations
4 Altmetric
Metrics details
In patients with chronic obstructive pulmonary disease (COPD), the maximum level of diaphragm excursion (DE max ) is correlated with dynamic lung hyperinflation and exercise tolerance. This study aimed to elucidate the utility of DE max to predict the improvement in exercise tolerance after pulmonary rehabilitation (PR) in patients with COPD.
This was a prospective cohort study. Of the 62 patients with stable COPD who participated in the outpatient PR programme from April 2018 to February 2021, 50 completed the programme. Six-minute walk distance (6MWD) was performed to evaluate exercise tolerance, and ultrasonography was performed to measure DE max . Responders to PR in exercise capacity were defined as patients who demonstrated an increase of > 30 m in 6MWD. The receiver operating characteristic (ROC) curve was used to determine the cut-off point of DE max to predict responses to PR.
Baseline levels of forced expiratory volume in 1 s, 6MWD, maximum inspiratory pressure, DE max and quadriceps muscle strength were significantly higher, and peak dyspnoea of modified Borg (mBorg) scale score was lower in responders (n = 30) than in non-responders (n = 20) to PR (p < 0.01). In multivariate analysis, DE max was significantly correlated with an increase of > 30 m in 6MWD. The area under the ROC curve of DE max to predict responders was 0.915, with a sensitivity and specificity of 83% and 95%, respectively, at a cut-off value of 44.9 mm of DE max .
DE max could adequately predict the improvement in exercise tolerance after PR in patients with COPD.
Chronic obstructive pulmonary disease (COPD) is a progressive disease characterised by minimally reversible airflow limitation [ 1 ]. The main feature of COPD is the inability of patients to cope with their activities of daily life due to shortness of breath. Although the pathophysiological mechanisms involved in the development of dyspnoea and poor exercise tolerance in patients with COPD are complex, dynamic lung hyperinflation (DLH) plays a central role [ 2 ] by increasing ventilatory workload and decreasing the pressure-generating capacity of the inspiratory muscles.
Pulmonary rehabilitation (PR) is a non-pharmacological intervention and has been reported to improve dyspnoea, exercise capacity and quality of life of patients with COPD [ 3 ]. Owing to a body of evidence, PR is now established as the standard of care for patients with COPD [ 4 ]. However, not all patients with COPD benefit from PR to the same extent. Therefore, identifying patients who are likely to achieve maximum benefit from the PR programme is crucial. So far, several studies have shown that severe airflow limitation or poor exercise tolerance at baseline may predict a better response to PR [ 5 , 6 ], but another study has reported inconsistent findings [ 7 ]. Furthermore, one study reported that patients with severe dyspnoea did not respond well to PR and patients with milder dyspnoea responded well [ 8 ].
Considering the role of DLH in the development of dyspnoea and poor exercise tolerance in patients with COPD, objective measures that reflect the degree of DLH may help in identifying good responders to PR. Previously, we reported that there was an association between increased dyspnoea due to DLH on exercise and decreased exercise capacity in patients with COPD and reduced mobility of the diaphragm, which was assessed by the maximum level of diaphragm excursion (DE max ) using ultrasonography [ 9 ]. Other research groups reported the utility of ultrasonographic assessment of diaphragmatic mobility in COPD in understanding its association with 6-min walk distance (6MWD), dyspnoea [ 10 ] and increased mortality [ 11 ].
However, there have been no reports on the association between diaphragmatic mobility and the effect of PR to improve exercise tolerance. The primary aim of this study is to clarify the role of DE max to predict the improvement in exercise tolerance after PR in patients with COPD.
Materials and methods
Study design and subjects.
This was a single-centre, observational, prospective cohort study. The study included 62 patients with clinically stable COPD who visited the Department of Respiratory Medicine and Allergology, Kindai University Hospital, between April 2018 and February 2021. The exclusion criteria included unstable medical conditions that could cause or contribute to breathlessness, such as metabolic, cardiovascular or other respiratory diseases, or any other disorders that could interfere with exercise testing, such as neuromuscular diseases or musculoskeletal problems. This study was approved by the Ethics Committee of Kindai University School of Medicine. Written informed consent was obtained from all participants.
Measurements
All participants underwent ultrasonography (Xario 200, Toshiba, Tokyo, Japan) for the assessment of their DE max . Using the liver as an acoustic window (Fig. 1 A), a convex 3.5 MHz probe was used to measure the excursions of the right hemidiaphragm according to the techniques mentioned in previous studies [ 9 , 12 , 13 ]. The M-mode cursor was rotated and placed on the axis of diaphragmatic displacement on the stored image, and displacement measurements were performed. Measurements were performed during each of the three deep breaths, and DE max was measured (Fig. 1 B). The maximum value obtained for the three deep breaths was used. 6MWD was performed to evaluate walking capacity according to the American Thoracic Society (ATS)/European Respiratory Society (ERS) statement [ 14 , 15 , 16 ]. All participants performed the 6MWD test before and after the PR programme, and the magnitude of their perceived breathlessness and their leg fatigue was rated using a 1–10-point Borg scale. Responders to PR in exercise capacity were defined as those who demonstrated more than 30 m increase in 6MWD after the PR programme, which was the definition of minimal clinically important difference (MCID) for 6MWD [ 17 ].
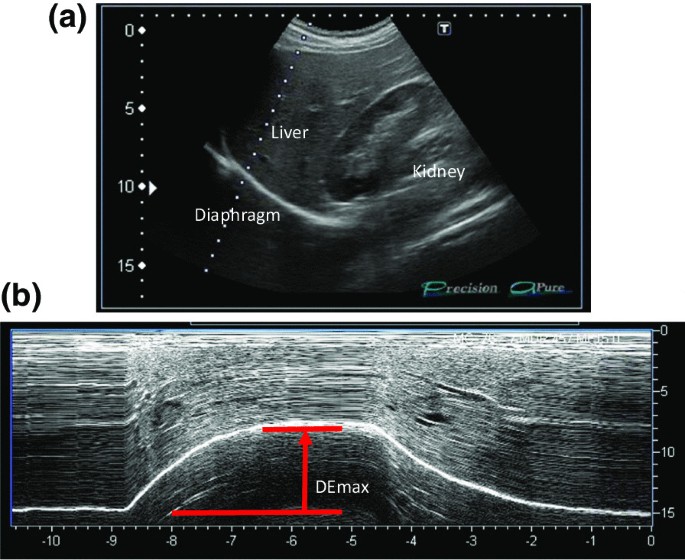
Representative image of the right diaphragm. The probe was positioned below the right costal margin between the midclavicular and anterior axillary lines. A Two-dimensional ultrasonographic image of the right hemidiaphragm (B-mode). Diaphragmatic movements were recorded in M-mode during deep breathing (DE max ) ( B )
Spirometry (CHESTAC-800, Chest, Tokyo, Japan) was performed following the 2005 ATS/ERS recommendations [ 18 ] for measuring forced vital capacity (FVC), forced expiratory volume in 1 s (FEV 1 ) and inspiratory capacity. Respiratory muscle strength was assessed by measuring the maximum inspiratory pressure (PI max ) generated against an occluded airway at residual volume [ 19 ] (SP-370, Fukuda Denshi, Tokyo, Japan). A hand-held dynamometer (μTasF-1, Anima Corp., Tokyo) was used to measure quadriceps muscle strength (QMS). The impact of COPD on health status was assessed using the COPD assessment test (CAT), a patient-completed questionnaire on eight items, namely, cough, phlegm, chest tightness, breathlessness, limited activities, confidence leaving home, sleeplessness and energy. The scores for each of the items range from 0 to 5 points, resulting in a CAT total score ranging from 0 to 40 points [ 20 ], and MCID of CAT is 2 points [ 21 ]. In all patients with COPD, emphysema was evaluated by computed tomography of the chest. A SYNAPSE VINCENT volume analyser (FUJIFILM Medical, Tokyo, Japan) was used to measure the low attenuation area (%LAA).
Rehabilitation programme
The outpatient PR programme was conducted twice a week for 12 weeks (24 sessions), including aerobic exercise training (ergometer and walking exercise) at 60–70% of peak workload for 20–40 min and upper- and lower-limb muscle strength training for 10–20 min.
Sample size
The sample size was estimated using R software. The analysis based on 6MWD data from the PR programme revealed that 40 subjects were required if the expected area under the curve (AUC) below the receiver operating characteristic (ROC) curve was 0.80, the power was 90%, and the significance level was 0.01. Furthermore, we anticipated a dropout from the PR programme. Thus, we set the sample size to 50 participants.
Statistical analysis
Responders and non-responders were compared using t -test, the Wilcoxon rank-sum test or χ 2 test, as appropriate. The paired t -test or the Wilcoxon signed-rank test was used to evaluate the changes in the parameters before and after the PR programme. The Pearson correlation coefficient was used to analyse the relationship between changes in 6MWD and independent variables because changes in 6MWD were normally distributed. Additionally, multivariate logistic regression models were used to assess the ability of variables to predict a response to PR. The ROC curve method was used to assess the ability of DE max to predict a response to PR. All statistical analyses were performed using the JMP software programme (JMP®, Version 14; SAS Institute Inc., Cary, NC, USA).
Out of the 62 patients included in the study, 50 completed the PR programme (Fig. 2 ). Two patients dropped out because of severe exacerbation of COPD, and 10 patients discontinued the PR owing to the coronavirus pandemic. Table 1 presents the baseline characteristics of the participants. After the PR programme, scores for CAT, 6MWD, peak dyspnoea and leg fatigue of the modified Borg (mBorg) scale, and QMS improved significantly (Table 2 ). Thirty patients showed an increase of > 30 m in 6MWD after PR (responders: 60%), and 20 patients (40%) were defined as non-responders. Baseline levels of %FEV 1 , 6MWD, PI max , DE max and QMS were significantly higher and those of CAT score and peak dyspnoea of mBorg scale were significantly lower in responders than in non-responders (Table 1 ). Changes in 6MWD were significantly correlated with baseline levels of CAT, %FEV 1 , peak dyspnoea of mBorg scale, PI max , DE max (Fig. 3 ) and QMS and marginally correlated with baseline levels of 6MWD (Table 3 ).
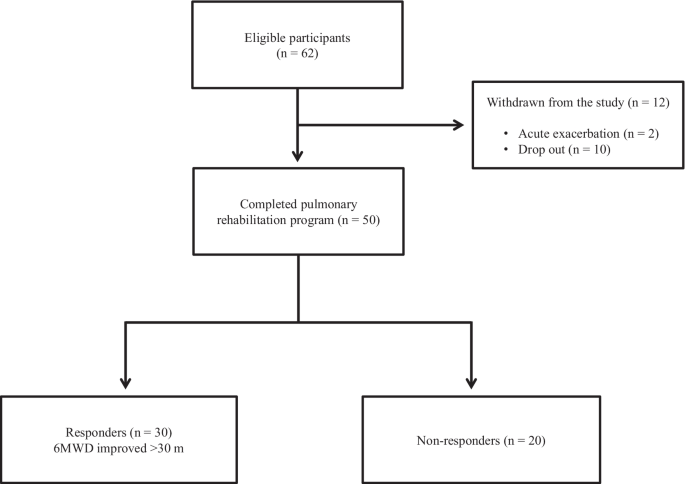
Study flow diagram. COPD chronic obstructive pulmonary disease, PR pulmonary rehabilitation, 6MWD 6-min walk distance
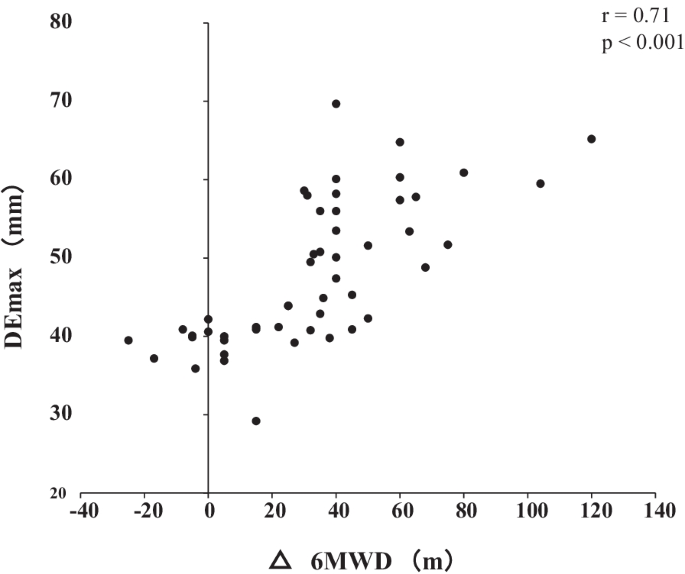
Relationship between DE max and the changes in 6MWD after pulmonary rehabilitation. Changes in 6MWD were significantly positively correlated with DE max (r = 0.72; p < 0.001). DE max maximum diaphragmatic excursion, 6MWD 6-min walk distance
In multivariate analysis, DE max alone significantly contributed to the prediction of responders (Table 4 , Model 1). When using PI max instead of DE max because PI max and DE max showed a strong association (r = 0.73), both PI max and %FEV 1 contributed to the prediction (Table 4 , Model 2). The area under the ROC curve of DE max to predict the responders was 0.915, with a sensitivity of 83% and a specificity of 95% at a cut-off value of 44.9 mm of DE max (Fig. 4 ). The significance of DE max in the predictability of responders remained even when the analysis was confined to severe patients (%FEV 1 < 50%, n = 23; AUC = 0.88, sensitivity = 70% and specificity = 100% at a cut-off value of 44.9 mm).

Receiver operating characteristic (ROC) curve for baseline DE max in relation to the response to pulmonary rehabilitation. ROC curve estimates the ability of DE max to predict a clinically important improvement in 6MWD (> 30 m) after pulmonary rehabilitation (AUC = 0.915, sensitivity = 83% and specificity = 95% at a cut-off point of 44.9 mm of DE max ). AUC area under the curve, 6MWD 6-min walk distance, DE max maximum diaphragmatic excursion
This is the first study to demonstrate the utility of DE max to predict the responsiveness of patients with COPD to 12-week PR. In this study, multivariate analysis revealed that greater baseline DE max was the only factor that predicted the responsiveness to PR, independent of baseline %FEV 1 . Additionally, the model using DE max had better prediction performance than that using PI max . The AUC of DE max to predict the 30 m or more improvement in 6MWD after the PR was 0.915, with a sensitivity of 83% and a specificity of 95% at 44.9 mm.
PR is beneficial to patients with chronic respiratory disease, including COPD [ 3 ], and generally improves exercise performance, health-related quality of life and dyspnoea [ 22 ], which was confirmed in this study. Ideally, PR was proven to be effective in all patients, but the response to PR varies considerably between individual patients [ 8 , 23 , 24 , 25 ]. Indeed, in this study, the improvement in 6MWD was less than that in MCID in 40% of the patients regardless of the degree of severity of COPD. Therefore, identifying predictors of a response is crucial in ensuring better PR efficacy and personalisation of PR programmes for patients with COPD.
In this study, the baseline values of %FEV 1 , PI max , DE max , QMS and 6MWD were positively associated with Δ6MWD in univariate analysis, suggesting that a better baseline condition was associated with a higher proportion of patients who achieved MCID after PR. These findings are consistent with those of previous studies that showed that patients with higher levels of %FEV 1 or FEV 1 /VC achieved greater improvement in 6MWD after PR [ 7 , 26 , 27 ] and a study in which patients with milder mMRC scores could achieve MCID of 6MWD after PR [ 8 ], but not for those with worst mMRC score, although others studies showed contradictory results [ 5 , 6 , 28 , 29 , 30 ] or found no significant baseline characteristics to predict a response to PR [ 31 ]. The discrepancy between the findings cannot be fully explained, but it might be due to the differences in the studied population and strength or length of PR. In this study, the mean %FEV 1 of the participants was 56.0%, which was relatively higher than that of other studies (mean %FEV 1 of 40–50% in most studies) [ 5 , 6 , 28 ], despite similar inclusion criteria throughout the studies, i.e., not limited to severe COPD in most studies. Thus, no ceiling effect with a PR programme that included high-intensity load exercise training for 20–40 min was observed in our population.
In this study, an important finding is that greater DE max at baseline was the only factor that predicted the responders in 6MWD after PR. In addition, the model using DE max had better prediction performance than that using PI max . The high predictability of DE max may be because of its strong association with DLH and dyspnoea during exercise, as reported previously [ 9 ]. DLH is involved in the development of dyspnoea, and both are important factors to determine the improvement in 6MWD in patients with COPD. Therefore, DE max that reflects the degree of DLH and dyspnoea during exercise was superior to other physiological indices to predict responders.
Furthermore, the virtuous cycle observed in our PR programme that included high-intensity load exercise training might be a result of the improvement in ventilation pattern. Improving the ventilation pattern would be easier with greater DE max , as shown in studies of mechanically ventilated patients [ 32 ], which may have reduced dyspnoea during exercise after 12 weeks of PR and improved exercise tolerance. Exercise therapy is a central component of PR, which significantly reduces blood lactate levels during exercise, reduces minute ventilation and improves exercise tolerance [ 33 ]. The high-intensity load exercise training, which is performed at 60–80% of the maximum oxygen uptake, has a higher physiological effect than low exercise load. Patients with greater DE max may be able to perform higher load training, which resulted in effective PR.
Diaphragm ultrasonography has been widely and successfully used to identify diaphragmatic dysfunction by showing its association with 6MWD, dyspnoea [ 10 ], extubation failure in mechanically ventilated patients [ 32 ], and increased mortality [ 11 ]. Recently, Lewinska and Shahnazzaryan proposed its use in pulmonary physiotherapy of patients with COPD [ 34 ]. In most previous studies, diaphragm ultrasonography was used to assess DE max , i.e., the measurement of the excursion of the right hemidiaphragm, as used in this study, and diaphragm thickness that assessed the length and thickness of the zone of apposition of the diaphragm against the rib cage [ 35 , 36 ]. However, it is difficult to measure diaphragm thickness in patients with severe COPD because the length of the zone of apposition is shorter in patients with COPD than that in control subjects [ 37 ], whereas it is easy to measure DE max, which shows high intra- and inter-observer reliability [ 38 ]. Bhatt et al. showed that improvement in 6MWD was associated with that in DE max during forced expiration when the effectiveness of pursed lips breathing was assessed in the PR of patients with COPD [ 39 ]. Corbellini et al. demonstrated greater improvement in DE max during inspiration after PR, which was associated with an increase in the inspiratory capacity [ 40 ]. The normal and cut-off values of DE max during normal respiration, forced respiration, and voluntary sniffing have been described for each gender [ 38 ]. Thus, DE max would be a useful and reliable measure for incorporation into the PR assessment. Furthermore, in clinical settings, this objective measure of DE max has additional advantages as it requires minimum effort in patients and can be applied to the PR programme at home if portable ultrasonography is used. However, the assessment of DE max has a limitation. The procedures pertaining to positioning of patients, breathing patterns, and the selected hemidiaphragm are not standardised at present, which may hamper the routine use of DE max at this moment. Standardisation of these parameters would further facilitate the use of DE max in clinical settings and for research purpose.
There are some limitations to this study. This was a single-centre study involving a relatively small number of participants, and their baseline condition might have been relatively preserved. Nonetheless, 46% of the participants showed FEV 1 < 50%, and the utility of DE max was also observed in these patients with severe airflow limitation. Furthermore, in this study, few patients discontinued the PR programme, except for patients who discontinued during the coronavirus pandemic, which indicates that there was no severe mismatch between the PR programme and the patients’ ability to successfully complete this programme. As another limitation, we did not evaluate any malnutrition factors, which could be an important determinant of diaphragmatic mobility. Nonetheless, DE max was a stronger predictor of the effectiveness of PR than other parameters, including QMS or lung function using multivariate analysis. Further studies with a large number of patients are required, and the utility of DE max should be examined in patients with the most severe form of COPD with a low-intensity load exercise programme.
In conclusion, DE max , which is a reliable and easy to perform measurement, could adequately predict the improvement in exercise tolerance after PR in patients with COPD. Assessment of DE max could aid in making medical decisions associated with therapeutic strategies.
Availability of data and materials
The datasets used and/or analysed during the current study are available from the corresponding author on reasonable request.
Abbreviations
Chronic obstructive pulmonary disease
Dynamic lung hyperinflation
- Pulmonary rehabilitation
6-Min walk distance
Minimal clinically important difference
Forced vital capacity
Forced expiratory volume in 1 s
Maximum inspiratory pressure
Quadriceps muscle strength
COPD assessment test
Low attenuation area
Area under the curve
Receiver operating characteristic
Modified Borg
Global initiative for chronic obstructive lung disease (gold). Global strategy for the diagnosis, management, and prevention of chronic obstructive pulmonary disease: 2020 report. . https://goldcopd.org/gold-reports/ last accessed: 20 Jan 2020.
Gagnon P, Guenette JA, Langer D, Laviolette L, Mainguy V, Maltais F, Ribeiro F, Saey D. Pathogenesis of hyperinflation in chronic obstructive pulmonary disease. Int J COPD. 2014;9:187–201.
Google Scholar
Spruit MA, Singh SJ, Garvey C, ZuWallack R, Nici L, Rochester C, Hill K, Holland AE, Lareau SC, Man WD, et al. An official American Thoracic Society/European Respiratory Society statement: key concepts and advances in pulmonary rehabilitation. Am J Respir Crit Care Med. 2013;188:e13-64.
Article PubMed Google Scholar
Dong J, Li Z, Luo L, Xie H. Efficacy of pulmonary rehabilitation in improving the quality of life for patients with chronic obstructive pulmonary disease: evidence based on nineteen randomized controlled trials. Int J Surg. 2020;73:78–86.
Boutou AK, Tanner RJ, Lord VM, Hogg L, Nolan J, Jefford H, Corner EJ, Falzon C, Lee C, Garrod R, et al. An evaluation of factors associated with completion and benefit from pulmonary rehabilitation in COPD. BMJ Open Respir Res. 2014;1:e000051.
Article PubMed PubMed Central Google Scholar
Costi S, Crisafulli E, Trianni L, Beghe B, Faverzani S, Scopelliti G, Chetta A, Clini E. Baseline exercise tolerance and perceived dyspnea to identify the ideal candidate to pulmonary rehabilitation: a risk chart in COPD patients. Int J Chron Obstruct Pulmon Dis. 2019;14:3017–23.
van Ranst D, Otten H, Meijer JW, van’t Hul AJ. Outcome of pulmonary rehabilitation in COPD patients with severely impaired health status. Int J Chron Obstruct Pulmon Dis. 2011;6:647–57.
Garrod R, Marshall J, Barley E, Jones PW. Predictors of success and failure in pulmonary rehabilitation. Eur Respir J. 2006;27:788–94.
Article CAS PubMed Google Scholar
Shiraishi M, Higashimoto Y, Sugiya R, Mizusawa H, Takeda Y, Fujita S, Nishiyama O, Kudo S, Kimura T, Chiba Y, et al. Diaphragmatic excursion correlates with exercise capacity and dynamic hyperinflation in COPD patients. ERJ Open Res 2020, 6.
Paulin E, Yamaguti WPS, Chammas MC, Shibao S, Stelmach R, Cukier A, Carvalho CRF. Influence of diaphragmatic mobility on exercise tolerance and dyspnea in patients with COPD. Respir Med. 2007;101:2113–8.
Yamaguti WPdS, Paulin E, Salge JM, Chammas MC, Cukier A, de Carvalho CRF. Diaphragmatic dysfunction and mortality in patients with COPD. J Bras Pneumol. 2009;35:1174–81.
Boussuges A, Gole Y, Blanc P. Diaphragmatic motion studied by m-mode ultrasonography: methods, reproducibility, and normal values. Chest. 2009;135:391–400.
Testa A, Soldati G, Giannuzzi R, Berardi S, Portale G, Gentiloni Silveri N. Ultrasound M-Mode assessment of diaphragmatic kinetics by anterior transverse scanning in healthy subjects. Ultrasound Med Biol. 2011;37:44–52.
Laboratories ATSCoPSfCPF. ATS statement: guidelines for the six-minute walk test. Am J Respir Crit Care Med. 2002;166:111–7.
Article Google Scholar
Holland AE, Spruit MA, Troosters T, Puhan MA, Pepin V, Saey D, McCormack MC, Carlin BW, Sciurba FC, Pitta F, et al. An official European Respiratory Society/American Thoracic Society technical standard: field walking tests in chronic respiratory disease. Eur Respir J. 2014;44:1428–46.
Singh SJ, Puhan MA, Andrianopoulos V, Hernandes NA, Mitchell KE, Hill CJ, Lee AL, Camillo CA, Troosters T, Spruit MA, et al. An official systematic review of the European Respiratory Society/American Thoracic Society: measurement properties of field walking tests in chronic respiratory disease. Eur Respir J. 2014;44:1447–78.
Polkey MI, Spruit MA, Edwards LD, Watkins ML, Pinto-Plata V, Vestbo J, Calverley PMA, Tal-Singer R, Agustí A, Bakke PS, et al. Six-minute-walk test in chronic obstructive pulmonary disease. Am J Respir Crit Care Med. 2013;187:382–6.
Miller MR, Hankinson J, Brusasco V, Burgos F, Casaburi R, Coates A, Crapo R, Enright P, van der Grinten CP, Gustafsson P, et al. Standardisation of spirometry. Eur Respir J. 2005;26:319–38.
Lisboa C, Munoz V, Beroiza T, Leiva A, Cruz E. Inspiratory muscle training in chronic airflow limitation: comparison of two different training loads with a threshold device. Eur Respir J. 1994;7:1266–74.
Jones PW, Harding G, Berry P, Wiklund I, Chen WH, Kline Leidy N. Development and first validation of the COPD Assessment Test. Eur Respir J. 2009;34:648–54.
Kon SSC, Canavan JL, Jones SE, Nolan CM, Clark AL, Dickson MJ, Haselden BM, Polkey MI, Man WDC. Minimum clinically important difference for the COPD Assessment Test: a prospective analysis. Lancet Respir Med. 2014;2:195–203.
Lacasse Y, Goldstein R, Lasserson TJ, Martin S. Pulmonary rehabilitation for chronic obstructive pulmonary disease. Cochrane Database Syst Rev 2006:CD003793.
Spruit MA, Gosselink R, Troosters T, Kasran A, Van Vliet M, Decramer M. Low-grade systemic inflammation and the response to exercise training in patients with advanced COPD. Chest. 2005;128:3183–90.
de Torres JP, Pinto-Plata V, Ingenito E, Bagley P, Gray A, Berger R, Celli B. Power of outcome measurements to detect clinically significant changes in pulmonary rehabilitation of patients with COPD. Chest. 2002;121:1092–8.
Troosters T, Gosselink R, Decramer M. Exercise training in COPD: how to distinguish responders from nonresponders. J Cardiopulm Rehabil. 2001;21:10–7.
Vagaggini B, Costa F, Antonelli S, De Simone C, De Cusatis G, Martino F, Santerini S, Paggiaro P. Clinical predictors of the efficacy of a pulmonary rehabilitation programme in patients with COPD. Respir Med. 2009;103:1224–30.
Scott AS, Baltzan MA, Fox J, Wolkove N. Success in pulmonary rehabilitation in patients with chronic obstructive pulmonary disease. Can Respir J. 2010;17:219–23.
Crisafulli E, Gorgone P, Vagaggini B, Pagani M, Rossi G, Costa F, Guarriello V, Paggiaro P, Chetta A, de Blasio F, et al. Efficacy of standard rehabilitation in COPD outpatients with comorbidities. Eur Respir J. 2010;36:1042–8.
Zanini A, Chetta A, Gumiero F, Della Patrona S, Casale S, Zampogna E, Aiello M, Spanevello A. Six-minute walking distance improvement after pulmonary rehabilitation is associated with baseline lung function in complex COPD patients: a retrospective study. Biomed Res Int. 2013;2013:1–6.
Ragaselvi S, Janmeja AK, Aggarwal D, Sidana A, Sood P. Predictors of response to pulmonary rehabilitation in stable chronic obstructive pulmonary disease patients: a prospective cohort study. J Postgrad Med. 2019;65:101–6.
CAS PubMed PubMed Central Google Scholar
Selzler A-M, Simmonds L, Rodgers WM, Wong EYL, Stickland MK. Pulmonary rehabilitation in chronic obstructive pulmonary disease: predictors of program completion and success. COPD J Chronic Obstr Pulm Dis. 2012;9:538–45.
Li C, Li X, Han H, Cui H, Wang G, Wang Z. Diaphragmatic ultrasonography for predicting ventilator weaning: a meta-analysis. Medicine (Baltimore). 2018;97:e10968.
Rabinovich RA, Ardite E, Troosters T, Carbo N, Alonso J, Gonzalezde Suso JM, Vilaro J, Barbera JA, Polo MF, Argiles JM, et al. Reduced muscle redox capacity after endurance training in patients with chronic obstructive pulmonary disease. Am J Respir Crit Care Med. 2001;164:1114–8.
Lewinska A, Shahnazaryan K. The use of diaphragm ultrasonography in pulmonary physiotherapy of COPD patients: a literature review. J Clin Med 2020; 9.
Gibson GJ, Whitelaw W, Siafakas N, Supinski GS, Fitting JW, Bellemare F, Loring SH, Troyer AD, Grassino AE. ATS/ERS Statement on respiratory muscle testing. Am J Respir Crit Care Med. 2002;166:518–624.
Summerhill EM, El-Sameed YA, Glidden TJ, McCool FD. Monitoring recovery from diaphragm paralysis with ultrasound. Chest. 2008;133:737–43.
McKenzie DK, Butler JE, Gandevia SC. Respiratory muscle function and activation in chronic obstructive pulmonary disease. J Appl Physiol. 2009;107:621–9.
Laveneziana P, Albuquerque A, Aliverti A, Babb T, Barreiro E, Dres M, Dubé BP, Fauroux B, Gea J, Guenette JA, et al. ERS statement on respiratory muscle testing at rest and during exercise. Eur Respir J 2019; 53.
Bhatt SP, Luqman-Arafath TK, Gupta AK, Mohan A, Stoltzfus JC, Dey T, Nanda S, Guleria R. Volitional pursed lips breathing in patients with stable chronic obstructive pulmonary disease improves exercise capacity. Chron Respir Dis. 2013;10:5–10.
Corbellini C, Boussuges A, Villafane JH, Zocchi L. Diaphragmatic mobility loss in subjects with moderate to very severe COPD may improve after in-patient pulmonary rehabilitation. Respir Care. 2018;63:1271–80.
Download references
Acknowledgements
Not applicable.
This work was supported by Grants-in-Aid for Scientific Research (21K11325).
Author information
Authors and affiliations.
Department of Rehabilitation Medicine, Kindai University School of Medicine, 377-2 Onohigashi, Osakasayama, Osaka, 5898511, Japan
Masashi Shiraishi, Yuji Higashimoto, Ryuji Sugiya, Hiroki Mizusawa, Yu Takeda, Shuhei Fujita, Tamotsu Kimura & Kanji Fukuda
Department of Respiratory Medicine and Allergology, Kindai University School of Medicine, Osaka, Japan
Masashi Shiraishi, Osamu Nishiyama, Yuji Tohda & Hisako Matsumoto
Inclusive Medical Science Research Institute, Morinomiya University of Medical Sciences, Osaka, Japan
Shintarou Kudo
Division of Biostatistics, Clinical Research Center, Kindai University School of Medicine, Osaka, Japan
Yasutaka Chiba
You can also search for this author in PubMed Google Scholar
Contributions
MS, YH, and YC made substantial contributions to the conception and design of the work. MS, YH, and RS made substantial contributions to the data acquisition. MS and HM made substantial contributions to the analysis. All of the listed authors designed the study and were involved in the interpretation of the data. MS and HM drafted the work. YH, MS, TK, YC, ON, KS, KF, YT, and HM revised the report critically for important intellectual content. All authors approved the final version to be published and agreed to be accountable for all aspects of the work. All authors read and approved the final manuscript.
Corresponding author
Correspondence to Masashi Shiraishi .
Ethics declarations
Ethics approval and consent to participate.
This study was approved by the Ethics Committee of Kindai University School of Medicine (31-086). Written informed consent was obtained from all participants.
Consent for publication
If the manuscript is accepted, we approve it for publication in Respiratory Research.
Competing interests
The authors declare no competing interests.
Additional information
Publisher's note.
Springer Nature remains neutral with regard to jurisdictional claims in published maps and institutional affiliations.
Rights and permissions
Open Access This article is licensed under a Creative Commons Attribution 4.0 International License, which permits use, sharing, adaptation, distribution and reproduction in any medium or format, as long as you give appropriate credit to the original author(s) and the source, provide a link to the Creative Commons licence, and indicate if changes were made. The images or other third party material in this article are included in the article's Creative Commons licence, unless indicated otherwise in a credit line to the material. If material is not included in the article's Creative Commons licence and your intended use is not permitted by statutory regulation or exceeds the permitted use, you will need to obtain permission directly from the copyright holder. To view a copy of this licence, visit http://creativecommons.org/licenses/by/4.0/ . The Creative Commons Public Domain Dedication waiver ( http://creativecommons.org/publicdomain/zero/1.0/ ) applies to the data made available in this article, unless otherwise stated in a credit line to the data.
Reprints and permissions
About this article
Cite this article.
Shiraishi, M., Higashimoto, Y., Sugiya, R. et al. Diaphragmatic excursion is correlated with the improvement in exercise tolerance after pulmonary rehabilitation in patients with chronic obstructive pulmonary disease. Respir Res 22 , 271 (2021). https://doi.org/10.1186/s12931-021-01870-1
Download citation
Received : 09 July 2021
Accepted : 15 October 2021
Published : 22 October 2021
DOI : https://doi.org/10.1186/s12931-021-01870-1
Share this article
Anyone you share the following link with will be able to read this content:
Sorry, a shareable link is not currently available for this article.
Provided by the Springer Nature SharedIt content-sharing initiative
- Diaphragmatic excursion
- Six-minute walk distance (6MWD)
Respiratory Research
ISSN: 1465-993X
- General enquiries: [email protected]
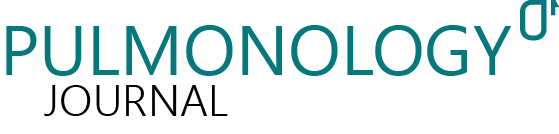
Pulmonology (previously Revista Portuguesa de Pneumologia) is the official journal of the Portuguese Society of Pulmonology (Sociedade Portuguesa de Pneumologia/SPP). The journal publishes 6 issues per year, mainly about respiratory system diseases in adults and clinical research. This work can range from peer-reviewed original articles to review articles, editorials, and opinion articles. The journal is printed in English, and is freely available in its web page as well as in Medline and other databases.

Indexed in:
Science Citation Index Expanded, Journal of Citation Reports; Index Medicus/MEDLINE; Scopus; EMBASE/Excerpta Medica
The Impact Factor measures the average number of citations received in a particular year by papers published in the journal during the two preceding years. © Clarivate Analytics, Journal Citation Reports 2022
CiteScore measures average citations received per document published.
SRJ is a prestige metric based on the idea that not all citations are the same. SJR uses a similar algorithm as the Google page rank; it provides a quantitative and qualitative measure of the journal's impact.
SNIP measures contextual citation impact by wighting citations based on the total number of citations in a subject field.
- Abbreviations
- Introduction
- Structure and function
- Diaphragmatic dysfunction
- Clinical presentation
- Radiography
- Fluoroscopy
- Pulmonary function tests
- Stimulation of the phrenic nerve
- Surgical diaphragmatic plication
- Phrenic nerve repair by microsurgery
- Diaphragmatic pacemaker
- Ventilatory support
- Author's contribution
- Conflict of interests
- Acknowledgement
- Bibliography
The diaphragm is the main breathing muscle and contraction of the diaphragm is vital for ventilation so any disease that interferes with diaphragmatic innervation, contractile muscle function, or mechanical coupling to the chest wall can cause diaphragm dysfunction. Diaphragm dysfunction is associated with dyspnoea, intolerance to exercise, sleep disturbances, hypersomnia, with a potential impact on survival.
Diagnosis of diaphragm dysfunction is based on static and dynamic imaging tests (especially ultrasound) and pulmonary function and phrenic nerve stimulation tests. Treatment will depend on the symptoms and causes of the disease. The management of diaphragm dysfunction may include observation in asymptomatic patients with unilateral dysfunction, surgery (i.e., plication of the diaphragm), placement of a diaphragmatic pacemaker or invasive and/or non-invasive mechanical ventilation in symptomatic patients with bilateral paralysis of the diaphragm. This type of patient should be treated in experienced centres.
This review aims to provide an overview of the problem, with special emphasis on the diseases that cause diaphragmatic dysfunction and the diagnostic and therapeutic procedures most commonly employed in clinical practice. The ultimate goal is to establish a standard of care for diaphragmatic dysfunction.
The diaphragm separates the thoracic cavity from the abdominal cavity. It is the main breathing muscle and is innervated by the phrenic nerves (PN) that arise from nerve roots C3–C5. Diseases that interfere with diaphragmatic innervation, contractile muscle properties, or mechanical coupling to the chest wall can cause diaphragmatic dysfunction. 1 Diaphragmatic dysfunction is associated with the presence of respiratory symptoms, especially dyspnoea, exercise intolerance, sleep disturbances, hypersomnia and, in the most severe cases, a negative impact on survival. 2
The purpose of this review was to identify the causes of diaphragmatic dysfunction, determine signs of the disease, and set diagnostic criteria for the establishment of a standard of care.
The diaphragm is the main breathing muscle. 2 During quiet inspiration, the dome shape of the diaphragm changes very little and the muscular action causes a shortening of the apposition zone (area in which the lower rib cage and the diaphragm are in direct contact) that makes the diaphragm to move caudally like a piston, thereby increasing abdominal pressure and decreasing pleural pressure. The latter is transmitted to the lung – causing it to insufflate – and the costal wall, which will tend to collapse. This action is compensated by an increase of abdominal pressure – which causes the thoracic cage to expand in the apposition area – and the contraction of the diaphragm in the lower ribs, which also opens the thoracic cage 2–4 ( Fig. 1 A and B).
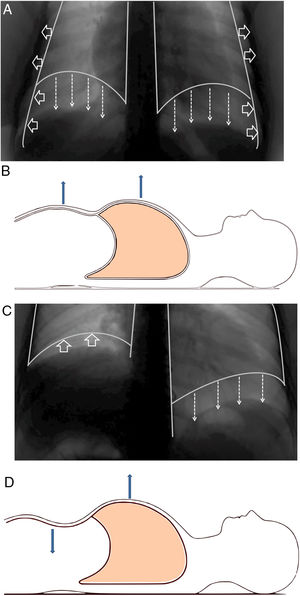
(A) Normal contraction of the diaphragm during quiet inspiration: the muscular action causes the diaphragm to move together like a piston in the caudal direction (direction of the arrows), thereby increasing abdominal pressure and decreasing pleural pressure. The latter is transmitted to the lung, causing it to be insufflated. (B) In supine position, it can be seen that both the rib cage and the abdomen move outwards. (C) When there is paralysis of the diaphragm (right side), the negative intrathoracic pressure drags the diaphragm and the abdominal viscera towards the thorax (direction of the arrows), which generates a negative abdominal pressure. (D) In supine position, it is observed how this negative abdominal pressure causes a paradoxical movement during inspiration: the abdomen moves inwards.
The term diaphragmatic dysfunction includes eventration, weakness and diaphragmatic paralysis. 5 Eventration is a permanent elevation of all or part of the hemidiaphragm caused by thinning. 5,6 Diaphragmatic weakness would be the partial loss of muscle strength to generate the necessary pressure for adequate ventilation, 6,7 while paralysis means the total absence of this capacity. This disorder, depending on the cause, can be unilateral or bilateral, temporary or permanent. 8 The hernia is the protrusion of an abdominal organ or tissue through a diaphragmatic defect. The most frequent congenital hernias are those of Bochdalek and Morgani 6 and, of those acquired, the hiatus hernia. 9 On chest X-ray they will be observed as a localized elevation of the diaphragm.
Another rare form of diaphragmatic dysfunction is diaphragmatic flutter. This dysfunction is characterized by the occurrence of repeated, variable-duration episodes of regular involuntary contractions. Signs of diaphragmatic flutter include pulsations in the epigastrium, dyspnoea and thoraco-abdominal pain. The aetiology of this condition is not well understood, and a standard of care has not been established yet. A set of trials have been performed with different agents, surgical ablation of the phrenic nerve and non-invasive ventilatory support, with varying results. 10,11
The incidence of diaphragmatic dysfunction is unknown, given the multiple diseases that cause it. The level of severity of this complication is determined either by the level of anatomical involvement or one-sidedness or bilaterality. 2,7,12 Fig. 2 shows by anatomical site the diseases that can cause diaphragmatic dysfunction from the cerebral cortex, through the internal capsule, the central nervous system, the spinal cord, the brachial plexus, the motor neurons and the PN, until reaching the neuromuscular synapse and the muscles themselves. 1,13–46 Table 1 shows its most relevant characteristics.
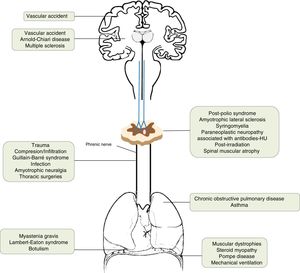
Causes of diaphragmatic dysfunction according to the level of involvement.
Causes that can cause diaphragmatic dysfunction.
Alterations such as hypokalemia, hypophosphatemia, hypomagnesemia or metabolic alkalosis; some connective tissue diseases, such as Shrinking lung syndrome (rare presentation of systemic lupus erythematosus that presents with respiratory distress and restrictive functional impairment); percutaneous punctures of veins (subclavian and internal jugular); placement of intercostal drainages; radiofrequency ablation; or chronic sclerosing mediastinitis, may also co-occur with diaphragmatic dysfunction. 2,7,42,47–52
Unilateral diaphragmatic dysfunction may be asymptomatic, 53 which explains why it is often diagnosed incidentally 5 when an elevation is observed in a hemidiaphragm on chest X-ray performed for another reason. Symptoms are usually more severe in obese patients or patients with an associated cardiac or pulmonary pathology. 2,7 The most frequent symptoms are dyspnoea on exertion and orthopnea, 53,54 but there may also be symptoms of nocturnal hypoventilation and gastroesophageal reflux. 55
Physical examination is non-specific: decreased respiratory sounds at the base of the affected hemithorax and possible dullness to percussion. 56 Paradoxical thoraco-abdominal movement during sleep occurs occasionally. Some studies have revealed that these patients tend to sleep with the healthy hemidiaphragm in the lower part. 57
When there is bilateral involvement, patients usually show symptoms of orthopnoea. Dyspnoea – which may occur at rest – becomes evident during immersion in water. 58 Patients usually show cyanosis, bilateral diminution of breathing sounds, rapid and superficial respiration, or paradoxical movement of the abdominal wall, 7,59 especially when the patient is in decubitus; 2,60 this is due to the “passive” behaviour of the diaphragm during inspiration. When the diaphragm is paralyzed, inspiration is obtained thanks to the contraction of the external intercostal muscles and accessory muscles (sternocleidomastoids, scalenes), which will expand the rib cage and generate intrathoracic negative pressure. This pressure will “drag” the diaphragm and abdominal viscera towards the thorax, which will generate a negative abdominal pressure and, therefore, a decrease in the anterior abdominal wall 60 ( Fig. 1 C and D). Most patients with diaphragmatic involvement have sleep disorders and significant hypoventilation, especially during REM sleep, with its related symptoms. 61,62 Table 2 shows the most relevant differences between unilateral or bilateral diaphragmatic paralysis.
Comparison of the clinical history, diagnostic tests and treatments according to diaphragmatic paralysis, either unilateral or bilateral (modified by McCool and Tzelepis) 7
MIP, maximum inspiratory pressure; NIV, non-invasive ventilation; Pdi max, transdiaphragmatic pressure after maximum inspiratory effort with closed glottis; TwichPdi, transdiaphragmatic pressure after transcutaneous electrical or magnetic stimulation of the phrenic nerve.
Patients with unilateral diaphragmatic dysfunction usually exhibit respiratory sleep disorders (fatigue, daytime sleepiness, snoring and apnea). Thus, some authors recommend that all patients with eventration or diaphragmatic paralysis undergo a full-night polysomnography. 63 Respiratory events generally include central hypopneas during REM sleep. These events often coincide with repeated episodes of desaturation that can be observed by pulse oximetry and are related to diaphragmatic weakness and paradoxical breathing. Desaturation is more frequent and severe when the patient is in lateral decubitus on the affected side. 64
Patients with bilateral diaphragmatic dysfunction show the same symptoms and desaturation events, although they are more likely to experience orthopnea. 62 The standard of treatment for patients with (unilateral or bilateral) diaphragmatic dysfunction and respiratory sleep disorders is continuous positive airway pressure or non-invasive mechanical ventilation. Yet, continuous positive airway pressure is more likely to fail in patients with bilateral diaphragmatic dysfunction, who will ultimately require non-invasive ventilation. Therefore, pressure titration should be performed in a sleep laboratory. 65
There is a range of potential pathophysiological mechanisms of hypercapnic respiratory failure in obese patients. Some of these mechanisms include diaphragmatic dysfunction secondary to the accumulation of adipose tissue and related mechanical alterations (inappropriate length-tension relationship 66 ). In the hypoventilation-obesity syndrome, the mechanisms that cause hypoventilation are complex and multifactorial. The role of diaphragmatic weakness in hypoventilation in obese patients is not well-understood; however, obesity seems to add an additional load to the respiratory system. 67
Suspicion of diaphragmatic dysfunction may arise from the study of unexplained dyspnoea or, occasionally, after the casual finding of a diaphragmatic elevation in an imaging test performed for another purpose. Whatever it is, diagnosis is usually based on imaging tests – both static and dynamic – including radiography, fluoroscopy and chest ultrasound. Table 2 summarizes the most relevant diagnostic tests for unilateral and bilateral diaphragmatic paralysis.
Chest X-ray is a simple effective test to evaluate the pulmonary parenchyma in search of other potential causes of dyspnoea. 12 X-ray allows physicians to see the structure, morphology and elevation of the diaphragm, has moderate interobserver reliability, and shows slightly more elevated values for the right hemidiaphragm. 68 Its sensitivity, specificity, positive and negative predictive value for the diagnosis of unilateral diaphragmatic paralysis are 90%, 44%, 33% and 93%, respectively. 68 However, in other studies, its sensitivity has not reached 70%. 69 In bilateral diaphragmatic paralysis, the typical finding is the elevation of the two hemidiaphragms, which is associated with small pulmonary volumes and bibasal atelectasis. 58 Although the presence of a diaphragmatic elevation is not necessarily a sign of dysfunction, its absence makes diaphragmatic dysfunction unlikely. 68
It is a test that allows us to visualize the diaphragm continuously throughout the normal respiratory cycle and during the execution of forced inspiratory manoeuvres. It is an easy-to-use and -interpret technique 5 with good inter-observer reliability 70 and, for years, it has been the gold standard for the diagnosis of diaphragmatic paralysis. 69 However, in some patients with bilateral diaphragmatic weakness, fluoroscopy findings can be misinterpreted, as some patients in the standing position may adopt an unusual respiratory pattern to compensate for their lack of mobility. 2,58 This mechanism of compensation may be misinterpreted in the fluoroscopy as a diaphragmatic contraction. 60 This situation can be prevented if the patient is in recumbent position, which is why some authors recommend that fluoroscopy is performed in this position. Therefore, fluoroscopy is a useful test for the diagnosis of unilateral hemidiaphragmatic paralysis. Conversely, fluoroscopy is not as useful for bilateral dysfunction, as findings can be misinterpreted. It should be performed with the patient in upright position (frontal and lateral) or in decubitus by an expert radiologist.
Diaphragmatic ultrasound is a non-invasive, portable, quick to perform, simple and well-tolerated test with a linear relationship between diaphragmatic movement and inspired volume, which allows quantitative and qualitative assessment of diaphragmatic movement. 71 Thus, ultrasound been suggested as the technique of choice for assessing diaphragmatic movement on suspicion of malfunctioning. 72 In addition, there is no exposure to ionizing radiation and intense patient cooperation is not essential. 69 In expert hands, and following the appropriate methodology, 73 it is a very reproducible technique, with good inter and intra-observer reliability and good reproducibility. 71–74 The thickness of the diaphragm can be determined in more than 85% of measurements, with a low coefficient of variation (0.09–0.14). 73 Variability of diaphragmatic movement can also be determined in virtually all measurements, with a good intra- and inter-observer correlation. 75,76 Ultrasound has shown to be useful for the detection of diaphragmatic dysfunction, 77 with a high sensitivity (93%) and specificity (100%) for diaphragmatic neuromuscular disease. 78 At present, many authors consider ultrasound the method of choice for the evaluation of diaphragmatic movement. 72,74,79
Hemidiaphragm visualization by ultrasound is achieved from an anterior approach, with the patient in supine position performing different breathing manoeuvres (quiet, deep breathing and sniff). Examination must start with the patient lying on the “healthy” side if unilateral paralysis is suspected. 56,69,75
The thickening of the diaphragm (Tdi) indicates a shortening of the diaphragm. Its absence during inspiration confirms diaphragmatic paralysis. If there is muscle atrophy, thickness decreases and the diaphragm does not contract during inspiration. 73,80 The lower limit of normal diaphragmatic thickness at rest (at the end of an unforced expiration) in most patients is 1.5 mm. 81 The two indexes usually used for the diagnosis of diaphragmatic paralysis include a Tdi value mm and a diaphragm thickening fraction (TFdi) value − thickness at the end of expiration)/thickness at the end of expiration (in %)]. 80 The normal lower limit accepted for the TFdi is 20%.
The normal movement of the diaphragm during inspiration is caudal, so the line corresponding to the diaphragm (echogenic line located between the liver or spleen and the lung) moves downward (approaching the transducer), preceded by a pause. Diaphragmatic paralysis shows an absence of caudal movement of the diaphragm during normal inspiration, or a paradoxical movement of the diaphragm during the sniff test and occasionally with deep inspiration. 5,69,82 Diaphragmatic weakness is determined where there is decreased amplitude of movement during deep breathing – with or without paradoxical movement during the sniff manoeuvre.
Pulmonary function tests are relevant to the diagnosis of diaphragmatic dysfunction. In general, weakness of the inspiratory muscles usually leads to a restrictive pattern, with a decrease in the total pulmonary, vital and functional residual capacities, keeping the CO diffusion and the residual volume preserved. The FEV 1 /FVC ratio is also relatively preserved. 54,57 The measurement of vital capacity is of great value. On the one hand, when vital capacity is normal, relevant inspiratory muscle weakness is unlikely. 83 On the other hand, a more severe decrease of 15–30% when going from the sitting position to decubitus – depending on whether paralysis is unilateral or bilateral – suggests some degree of diaphragmatic weakness and requires further examination. 2
One way of estimating the strength of respiratory muscles is by measuring the pressures they generate at different points in the airway. To do this, two types of tests can be used: (a) non-invasive tests, which determine the pressures generated in the mouth, nose or endotracheal tube; 84 and (b) invasive tests, which require the placement of pressure probes in the stomach and/or oesophagus that will serve as a reflex of abdominal and pleural pressure, respectively.
Determination of maximum static pressures in the mouth during inspiration (MIP) and expiration (MEP) with the airway closed is considered a reasonable method for measuring the force generated jointly by the inspiratory and expiratory muscles. In addition, it is one of the most widely used techniques in clinical practice. This technique is easy to perform and well tolerated. 85 Its greatest disadvantage is that it is highly dependent on the cooperation and effort of the patient. 86 In general, absolute values of MIP above 80 cm H 2 O in men and 70 cm H 2 O in women exclude clinically relevant inspiratory muscle weakness. 83 Normal MEP combined with low MIP suggests the existence of isolated weakness of the diaphragm. 85 Finally, the concomitant reduction of MIP and MEP suggests that diaphragmatic involvement may be due to a generalized process, with simultaneous involvement of the inspiratory and expiratory muscles. 7 In percentage values, MIP is around 60% of the predicted value (on average) in unilateral affectation 87 vs. 40% in bilateral dysfunction. 88 Nonetheless, a diminished MIP is not exclusive to muscular weakness and can be observed in patients with chronic obstructive pulmonary disease. 89
The nasal sniff manoeuvre is used do determine inspiratory pressures in the nose and involves the performance of a rapid voluntary inspiratory effort through the nasal passages. It is a useful test for evaluating the strength of the diaphragm in clinical practice. 85 A pressure, in absolute values, greater than 70 mm Hg in men and 60 mm Hg in women is unlikely to be associated with significant inspiratory muscle weakness. 85
The most widely used invasive tests include oesophageal pressure (Pes) and transdiaphragmatic pressure (Pdi) measurement by estimating the difference between Pes (intrathoracic pressure) and gastric pressure (Pga) (intra-abdominal pressure) [Pdi = Pes − Pga]. Pes and Pdi can be obtained during maximum voluntary efforts, the most frequent being the sniff test (Sniff Pdi). Pdi is specific to diaphragm contraction and is the gold standard method for the evaluation of diaphragm function. Also, Pdi is the only reliable diagnostic method for bilateral paralysis. 89 If an inspiratory effort is made with the paralyzed diaphragm, Pes and Pga will be negative and, therefore, the Pdi will not change. 85 In clinical practice, Sniff Pes and Sniff Pdi are the two most reproducible voluntary tests for assessing overall respiratory and diaphragmatic force. 83 A value of Sniff Pdi > 100 cm H 2 O in men and 80 cm H 2 O in women make the existence of clinically significant diaphragmatic weakness unlikely. 85 A Pdi of 0 confirms bilateral diaphragmatic paralysis 89 although some authors have established the cut-off point at mm Hg.
The gold standard method for the quantification of the mechanical function of the diaphragm is by measuring the negative pressure generated by its contraction in response to the stimulation of the PN. 85 This method offers the possibility of activating and studying the diaphragm separately without the activation and concomitant action of other muscle groups. During stimulation, negative pressure can be monitored by calculating the difference between oesophageal and gastric pressures (Twitch Pdi). Transcutaneous electrical phrenic stimulation can be performed at the level of the neck unilaterally or bilaterally. However, this technique causes the patient discomfort and is technically more difficult in obese patients or in patients with anatomical alterations. The magnetic stimulation of PNs is usually applied bilaterally at the level of the cervical spine 90 ; it is reproducible, easy to perform and well tolerated by patients. A Twitch Pdi 10–20 cm H 2 O (depending on whether involvement is unilateral or bilateral) is generally suggestive of diaphragmatic dysfunction. 91 Measuring Sniff Pdi and Twitch Pdi allows differential diagnosis of diaphragmatic paralysis caused either by first or second motor neuron involvement, a central cause, or lack of cooperation. 92
Although electromyography and the stimulation test must be performed by experienced operators, they are very accurate in the assessment of neural and muscular disorders.
Electromyography is performed by the insertion of a needle electrode. This test can show abnormal spontaneous activity of the diaphragm, and it can also show different characteristics of motor unit potential, like amplitude, shape or recruitment. 93 The uses of electromyography in the examination of respiratory muscles are described in specific guides. 85
Findings in electromyography are supported by evidence obtained in other functional tests – such as PN conduction studies. Electromyography is a very useful method for determining the diagnosis, evolution and prognosis of PN disorders. Although electromyography is associated with potential complications, it has been demonstrated to be safe. 94
Stimulation tests measure the efficacy of neural and neuromuscular transmission. They can be performed using electrical or magnetic stimulators. Electrical stimulators are less expensive and relatively selective but they cause the patients discomfort and the technique is complex. Magnetic stimulators are easy to use and cause less discomfort, but they are less selective and more expensive.
PN is stimulated at the level of the neck, and the electromyographic activity of the diaphragm is registered to measure PN latencies and amplitudes of muscle compound action potentials. In some neuromuscular disorders (i.e., demyelinating polyneuropathies), latencies are delayed due to slow PN conduction (6–8 ms in healthy adults). In other settings (PN trauma) the amplitude of muscle action potentials can be decreased (normal amplitude values average 500–800 mV). A lack of muscle action potential after phrenic stimulation is suggestive of diaphragmatic paralysis with a lesion near or at the neuromuscular junction. Cortical stimulation is usually performed using a magnetic stimulator to measure response time of the diaphragm. This time is compared with latency after direct stimulation of the PN, which yields central conduction time. Cortical stimulation is not selective and its application to the respiratory system is difficult. 85
The treatment of diaphragmatic paralysis depends mainly on its cause and the symptomatology of the patient. In general, patients with asymptomatic unilateral involvement do not require treatment. Initially, all associated factors must be treated, including obesity, respiratory or chronic heart diseases, which could influence and increase the symptoms of paralysis. There are specific treatments when the aetiology of the paralysis is known and is potentially reversible, as in infectious processes, 34,91 metabolic, endocrinological (such as diabetes 94 or hypothyroidism 95 ) or systemic erythematosus lupus (shrinking lung syndrome). 47 We must also bear in mind that paralyzes of idiopathic cause – such as amyotrophic neuralgia – can resolve spontaneously. 26,44 Other studies have shown that diaphragmatic paralysis of potentially reversible aetiology (surgical, paraneoplastic, diabetic neuropathy, etc.) can improve spontaneously the strength of the diaphragm and respiratory muscles by 40–60% of cases over time, 96–98 suggesting the convenience of delaying any surgical approach.
During the observation period, the patient can be included in a specific respiratory rehabilitation plan. 99 It has been shown that one-year inspiratory muscle training after cardiac surgery improves diaphragmatic mobility and the inspiratory muscle strength of patients with diaphragmatic dysfunction. 100
This is the main surgical correction treatment available to control dyspnoea in patients with diaphragmatic paralysis. It consists of folding the paralyzed diaphragm so that it is immobilized in a position of maximum inspiration, thereby relieving compression of the lung parenchyma and allowing lung reexpansion. It can be done through a thoracic (with thoracoscopy) 99 or abdominal approach. 101 It is primarily indicated for symptomatic patients with unilateral diaphragmatic dysfunction that – based on clinical, radiological and functional tests – has not resolved after a period of observation of 6–12 months and is therefore considered permanent and irreversible. 99,102 Plication has also been successfully performed in some patients with bilateral involvement. 102,103 In the series of patients operated on, the main causes of paralysis were traumatism, cardiac surgery and iatrogenic. 99,104
Plication has been shown to be effective, safe and cause few complications, 99,104–106 inducing an improvement of symptoms and dyspnoea. 100,105 The beneficial effects of plication are not only visible on radiological scans 101,104 but also in improved pulmonary function parameters. 99,102,104,105 After surgery, improvements occur in the tidal volume of both hemidiaphragms (the operated and the healthy, probably related to a significant improvement in the expansion of the abdominal compartments of the rib cage), 107 exercise capacity, 108 daily activity and quality of life, with a reduction of up to 20 points in the score on Saint George's Respiratory Questionnaire. 99,101,108 All this allows many patients to return to normal life. Morbid obesity, calcification of the diaphragm and certain neuromuscular diseases are relative contraindications. 109
This surgical approach – which includes modalities such as local decompression, transposition or interposition of a nerve graft – can be indicated for patients with unilateral phrenic involvement of a mainly iatrogenic or traumatic origin who have not shown any clinical or radiological improvement in a reasonable period of time. It is necessary to previously demonstrate the continuity of the nerve and the viability of the neuromuscular plate through PN conduction studies and electromyography. 110
It can be placed in patients with impaired bilateral mobility of the diaphragm who wish to delay the initiation of ventilation – both invasive and non-invasive – or who have started it but do not wish to continue or were not able to tolerate it. These patients generally exhibit cervical involvement at a level above C3, or with central alterations different from cervical involvement, – mainly congenital or acquired central hypoventilation. It can also be seen in patients with lower motor neuron involvement for a reason other than amyotrophic lateral sclerosis 111 and in traumatological or idiopathic etiologies. 112
The most relevant studies published so far on the use of a diaphragmatic pacemaker in amyotrophic lateral sclerosis have not confirmed its expected benefits, with higher mortality rates in patients using a pacemaker. Therefore, at present, it is not indicated for this type of patients. 113,114 The patients to whom this treatment is offered must be strictly selected and studied in institutions with experience; the presence of severe nocturnal hypoventilation must be confirmed and PN, diaphragm, and lung function must be shown to be ideal. 111
It has been used successfully both in patients with unilateral and bilateral diaphragmatic paralysis, either permanently in the latter, 115 or temporally in the former, until complete recovery of diaphragmatic function. Ventilatory support can be applied by invasive mechanical ventilation or non-invasive positive pressure ventilation (NPPV). NPPV is actually considered the tool of choice mainly in symptomatic patients with bilateral diaphragmatic paralysis. Tolerance is good, 116 and it has been shown to provide both clinical and blood gas improvement in the long term. 117 The indication of non-invasive ventilation would be similar to that for other neuromuscular or restrictive pathologies. 118,119
Patients with acute respiratory failure may need intubation and mechanical ventilation, which can continue over time as a result of respiratory muscle paralysis. A study in 152 patients with spinal cord injury (50% with affectation at C3–C5 level) revealed that early tracheostomy reduces the duration of invasive ventilation and length of stay in the ICU; in addition, it decreases the incidence of complications associated with orotracheal intubation, except for ventilation-associated pneumonia. 120 However, non invasive ventilation has been proposed as a weaning method prior to tracheostomy in collaborative patients with bilateral diaphragmatic paralysis, a small volume of secretions and an appropriate inspiratory flow. 115
Tracheostomy and invasive ventilation can also be required by patients with neuromuscular disease when non-invasive ventilation has failed or invasive interventions are ineffective. 121
Non-invasive ventilation is associated with some complications. Mild or transient complications are related to the use of masks. Severe complications can be caused by: (1) ventilation failure, which can be minimized by the strict selection of patients and the appropriate control of ventilation; (2) ventilation-associated pneumonia, with a lower risk in patients on invasive ventilation; (3) barotraumas, with a lower incidence than in patients on invasive ventilation; and (4) hypotension. 122
The diagnostic and therapeutic algorithms for unilateral and bilateral diaphragmatic paralysis are shown in Fig. 3 A and B, respectively.
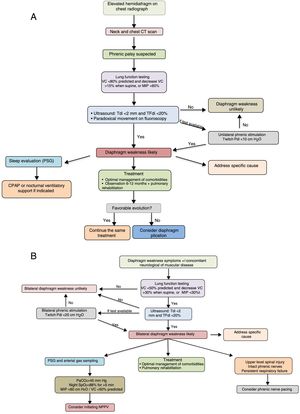
Suggestions for diagnostic and therapeutic algorithms in unilateral (A) and bilateral (B) diaphragmatic paralysis (modified from Dubé and Dres 12 ). CPAP, continuous positive pressure in the airway; CT, computed tomography; GSA, arterial blood gases; MIP, maximum inspiratory pressure; NIV, non-invasive ventilation; Pdi, transdiaphragmatic pressure; NPSG, nocturnal polysomnography; SaO 2 , arterial oxygen saturation; TFdi, fraction of thickening of the diaphragm; VC, vital capacity.
In summary, diaphragmatic dysfunction can be associated with important clinical consequences. Identifying its origin and treating its symptoms and effects on sleep structure and exercise capacity requires thorough examination. Ultrasound is a simple and effective means of routinely assessing diaphragm function which guides clinicians in their therapeutic choice. Diaphragmatic dysfunctions should be treated in experienced centres, with access to diaphragmatic ultrasonography, phrenic stimulation, pacemaker placement, and surgical experience in diaphragmatic plication.
Jorge Ricoy. Author. Conception and design. Writing of the article presented. Approval of the final version.
Nuria Rodríguez-Núñez. Co-author. Review of the submitted article. Approval of the final version.
José Manuel Álvarez-Dobaño. Co-author. Review of the submitted article. Approval of the final version.
María E. Toubes. Co-author. Review of the submitted article. Approval of the final version.
Vanessa Riveiro. Co-author. Review of the submitted article. Approval of the final version.
Luis Valdés. Author. Responsible. Conception and design. Writing of the article presented. Approval of the final version.
The authors declare the non-existence of external financing of this article.
We wish to confirm that are no known conflicts of interest associated with this publication and there has been no financial support for this work that could have influenced its outcome.
The authors would like to thank José Ángel Novo-Platas for his help with the figures.
- Subscribe to our newsletter
- Send to a friend
- Export reference
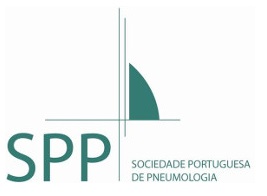
- Instructions for authors
- Submit an article
- Ethics in publishing
- Language Editing services
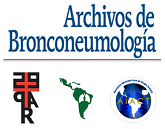
- Articles in press
- Current Issue
- Supplements
- Aims and scope
- Editorial Board
- Advertising
- Most often read
- Most popular
- All metrics
- Open access
- Download PDF
Are you a health professional able to prescribe or dispense drugs?

- Students & Professionals
Guide to Diaphragmatic Weakness & Paralysis
A weak or paralyzed diaphragm often goes misdiagnosed and left untreated, causing breathing issues that can worsen over time. While there are several medical treatments options, surgery remains the most effective way to treat a paralyzed or weakened diaphragm.
What is the Diaphragm?
The diaphragm is a large muscle that sits below the lungs and heart. The diaphragm is important as it is the primary muscle that facilitates breathing. When the diaphragm contracts, it becomes smaller, causing the lungs to expand in the chest cavity and allowing air to move into the lungs (inhaling). When it relaxes, it enlarges, causing a decrease in lung size thus forcing air out (exhaling).
The diaphragm is controlled by the phrenic nerve, a nerve that is attached to the cervical spine, the area of the spinal cord found in your neck. A paralyzed diaphragm is rarely caused by an injury to the diaphragm itself, but rather by an injury to the phrenic nerve or cervical spine.
What is Diaphragm Weakness or Paralysis?
Patients with a paralyzed diaphragm experience weakness of the diaphragm and have reduced breathing capabilities or are unable to control their voluntary breathing. They also have difficulty maintaining adequate gas exchange, as the lungs are not able to inhale and exhale outside air as efficiently. This is because the phrenic nerve is sending weak signals to the diaphragm to relax or contract, or is unable to send any signal part of or the entire diaphragm.
Diaphragm paralysis can be unilateral or bilateral .
Unilateral paralysis involves one side of the diaphragm. This means that the diaphragm is partially functioning, and the part that is paralyzed will move higher into the chest cavity, taking up space meant for the lungs and interfering with breathing.
Bilateral paralysis occurs when the entire diaphragm is paralyzed. This means that the diaphragm is unable to function in inhalation and exhalation and often requires a machine to assist with breathing.
What Causes Diaphragm Paralysis and Weakness?
Diaphragmatic weakness or paralysis is caused by damage or pressure on the phrenic nerve. There are several known causes that can lead to diaphragm paralysis:
- Birth defects such as congenital central hypoventilation syndrome
- Diseases of the nervous system, such as amyotrophic lateral sclerosis (ALS) or multiple sclerosis
- Injury, such as an upper cervical spinal cord injury that has spared the phrenic nerve
- Phrenic nerve fraying or damaging following cardiothoracic or pulmonary surgery
- Cervical spine arthritis
- Cancer that has spread and compresses the phrenic nerve.
While the cause can be identified in some cases, as many as 40 to 50% of paralyzed diaphragms are idiopathic, meaning the cause is unknown.
What are the symptoms of diaphragmatic weakness and paralysis?
Because the diaphragm plays a central role in breathing, the symptoms are more apparent when both sides of the diaphragm are affected. Symptoms of bilateral diaphragmatic weakness and paralysis include:
- Difficulty breathing, both at rest and when active
- Difficulty sleeping
- Recurrent pneumonia
In unilateral diaphragmatic paralysis, which affects only one side of the diaphragm, dyspnea – or difficulty breathing – on exertion or when lying down is the most typical symptom.
How are diaphragmatic weakness and paralysis diagnosed?
Sometimes diaphragmatic weakness and paralysis is an incidental finding with imaging such as x-rays, MRIs, or ultrasounds. Other times, the diagnosis can be made with blood tests, such as arterial blood gas analysis, that can reveal a lack of needed oxygen in the bloodstream. Pulmonary function tests may also be performed to help confirm diaphragm issues.
What is the treatment for diaphragmatic weakness?
Depending on the severity of injury to the diaphragm, some doctors recommend non-surgical options to treat the breathing issues associated with diaphragm weakness and paralysis. Surgical treatment is an option for more advanced cases or if breathing becomes so impacted daily life is affected.
- Diaphragmatic Pacing : If the phrenic nerve is intact, diaphragmatic pacing is an option. Diaphragmatic pacing is a minimally-invasive surgical option that involves placing a pacemaker to regulate breathing by electrically stimulating the phrenic nerve.
- Diaphragm Plication : Plication is another surgical option for treating a paralyzed diaphragm. Plication involves tying the affected side of the diaphragm to the side that is still functioning normally. This prevents the weakened diaphragm from becoming elevated in the chest cavity and allows the lungs to expand more efficiently and making breathing easier.
Mechanical ventilation with a breathing machine might be required in some more advanced cases.
If you need help for a diaphragm issue, we’re here for you. Call (212) 305-3408 for existing patients, (212) 304-7535 for new patients, or request an appointment online to get started today.
Related Services
- Diaphragm Center See all Related Services
Related Topics
- Diaphragm eventration
- Diaphragmatic hernias
- Diaphragmatic Pacing See all Related Topics
- Research article
- Open access
- Published: 27 January 2023
Clinical values of diaphragmatic movement in patients with chronic obstructive pulmonary disease
- Taehwa Kim 1 , 2 na1 ,
- Sungchul Huh 3 na1 ,
- Jae Heun Chung 1 , 2 ,
- Yun Seong Kim 1 , 2 ,
- Ra Yu Yun 3 , 4 ,
- Onyu Park 5 &
- Seung Eun Lee ORCID: orcid.org/0000-0002-4266-7722 1 , 2
BMC Pulmonary Medicine volume 23 , Article number: 33 ( 2023 ) Cite this article
2594 Accesses
3 Citations
1 Altmetric
Metrics details
The limitation of activity due to dyspnea in chronic obstructive pulmonary disease (COPD) patients is affected by diaphragmatic dysfunction and reduced lung function. This study aimed to analyze the association between diaphragm function variables and forced expiratory volume in the first second (FEV1) and to estimate the clinical significance of diaphragm function in the correlation between COPD severity and lung function.
This prospective, single-center, cross-sectional observational study enrolled 60 COPD patients in a respiratory outpatient clinic. Data for baseline characteristics and the dyspnea scale were collected. Participants underwent a pulmonary function test (PFT), a 6-minute walk test (6MWT), and diaphragm function by ultrasonography.
The right excursion at forced breathing showed the most significant correlation with FEV1 ( r = 0.370, p = 0.004). The cutoff value was 6.7 cm of the right diaphragmatic excursion at forced breathing to identify the FEV1 above 50% group. In the group with a right diaphragmatic excursion at forced breathing < 6.7 cm, modified Medical Research Council (mMRC), St. George's Respiratory Questionnaire and the total distance of 6MWT showed no difference between groups with FEV1 under and above 50% ( p > 0.05). In the group with ≥ 6.7 cm, mMRC and the total distance of 6MWT showed a significant difference between FEV1 under and above 50% ( p = 0.014, 456.7 ± 69.7 m vs. 513.9 ± 60.3 m, p = 0.018, respectively).
The right diaphragmatic forced excursion was closely related to FEV1, and analysis according to the right diaphragmatic forced excursion-based cut-off value showed a significant difference between both groups. When the diaphragm function was maintained, there was a lot of difference in the 6MWT’s factors according to the FEV1 value. Our data suggest that diaphragmatic function should be performed when interpreting PFT.
Peer Review reports
Introduction
The most common complaint in respiratory diseases regardless of the disease type is dyspnea [ 1 ]. COPD is characterized by worsening dyspnea during movement [ 2 ]. COPD restricts various activities of daily living due to shortness of breath, leading to poor quality of life and increased mortality and morbidity [ 3 ]. There are many causes of dyspnea; however, for patients with stable COPD, a major contributor is the weakening of the respiratory muscles, excluding conditions such as acute infectious diseases [ 4 ].
The diaphragm is the main respiratory muscle, particularly the inspiratory muscles. The weakness of the diaphragm in COPD has been extensively studied. Some studies have reported a significant reduction in diaphragmatic excursion in patients with COPD [ 5 , 6 , – 7 ]. Lung hyperinflation-associated shortening of the diaphragm has traditionally been considered a major cause of diaphragmatic weakness [ 8 ]. Also, there were previous studies about diaphragmatic thickness. Diaphragmatic thickness was a factor related to weaning and prognosis in patients under mechanical ventilation [ 9 , 10 ]. Recently, several studies have reported the clinical value of diaphragm ultrasonography according to COPD severity, and even compared to traditional methods, the diagnostic value of ultrasonography has proven to be reliable and useful [ 11 ]. Ultrasonography is also commonly used in medical facilities because it can be carried out anywhere, has no associated radiation risk, and can be used to adequately visualize the structure of the diaphragm [ 12 ].
Furthermore, 6MWT is an important tool for assessing exercise capacity and functional status in patients with COPD. Diaphragmatic weakness can impair physical performance, especially the 6MWT [ 13 , 14 ]. A previous study reported that pulmonary function was significantly correlated with the 6MWT in patients with severe and very severe COPD [ 15 ]. The relationship between 6MWT and PFT is a matter of connecting and understanding the respiratory muscles. PFT is used to measure the volume and flow rate of the lungs, and 6MWT is an important test for evaluating the exercise capacity and functional status of patients.
When we summarize the above, PFT correlates with 6MWT in COPD patients [ 15 ]. 6MWT can evaluate physical performance of COPD patients. Physical performance can also reflect diaphragmatic weakness [ 13 , 14 ]. Therefore, PFT correlates with 6MWT, 6MWT reflects physical performance, and physical performance was associated with diaphragmatic weakness. This relationship of PFT and diaphragmatic weakness can be expressed as follows for the patient. If the pulmonary function expressed by PFT is good, or if case which the power and strength of the respiratory muscles are good when PFT remains the same, breathing is more stable. Therefore, understanding the physiological principles of the respiratory muscle performance that establish the relationship these and compensate for this is important for managing the patient’s condition. Through this study, a review of the correlation between the PFT reflecting the 6MWT and diaphragm ultrasound features of respiratory muscle may be helpful to understand the physiological principles of patients with COPD.
Thus, this study aimed to analyze diaphragm movement characteristics using ultrasonography in patients with COPD and clarify its association with pulmonary function.
Study design and methods
Study design and participants.
This single-center, prospective, cross-sectional observational study recruited participants from a tertiary hospital outpatient respiratory clinic between April 2020 and April 2021. The inclusion criteria were: 1) patients 18 years old or older diagnosed with COPD by a pulmonologist; COPD diagnostic criterion was a post-bronchodilator FEV1/forced vital capacity (FVC) ratio < 0.70 based on the Global Initiative for Chronic Obstructive Lung Disease (GOLD), 2) patients who could maintain the required posture for diaphragm function measurement by ultrasonography and stable breathing during the examination such as 6MWT. Patients unable to cooperate with the examination and unstable patients requiring immediate medical intervention were excluded. Patients with interstitial lung disease featured on chest computed tomography (CT) that could affect diaphragm movement were also excluded.
Sixty-nine patients were enrolled, six of whom with combined interstitial lung disease on CT were excluded. Two patients were lost to follow-up, and one died before all examinations were completed. Finally, 60 patients completed all examinations for the study protocol and were included in the analysis.
All patients provided informed consent before participating in the study. Each patient’s clinical information was collected from four domains: pulmonary function, exercise capacity, body composition, and diaphragm function. Pulmonary function was evaluated through spirometry, MIP, and maximal expiratory pressure (MEP). Exercise capacity and body composition were assessed using the 6MWT and bioelectrical impedance analysis (BIA). Diaphragm dysfunction is defined as loss of muscle contractility [ 16 ]. To evaluated diaphragm dysfunction, we was assessed using ultrasonography in both the M-mode and B-mode for excursion and thickness, respectively.
Assessments
For patients who had performed a PFT within 1 month of participating in the study, the previous results were used and no retesting was performed. Patients who had no available PFT results within 1 month of participating in this study were reevaluated after enrollment. The Carefusion Vmax 20 (VIASYS Healthcare Inc. Sensormedics; Yorba Linda, CA, USA) was used for PFTs and FEV1, FVC, diffusing capacity of the lungs for CO, and total lung capacity were measured using the body plethysmography test. Regarding spirometry, the patients sat in a small booth and breathed into a mouthpiece. One technical expert from the Department of Respiratory Medicine conducted all the tests to maintain the consistency of the results.
MIP (PONY FX, COSMED Inc.; Rome, Italy) and MEP (PONY FX, COSMED Inc.; Rome, Italy) were measured in the sitting position using a portable mouth pressure meter. Three consecutive MIP and MEP measurements were taken, and the best result was recorded. The PFT was measured in a sitting position. A flanged mouthpiece was applied to the short and rigid tube of the measuring instrument and air leakage was checked around the mouthpiece before testing. The test was performed by an experienced examiner who has conducted the test for more than 8 years. MIP was measured by exhaling as deep as possible and inhaling as hard as possible for at least 1.5 s. MEP was measured by inhaling as deep as possible and exhaling as hard as possible for at least 1.5 s. Both measurements were made three times, and patients recovered to normal breathing patterns with at least a minute of break between measurements. The highest of the three measurements was recorded [ 17 ].
The 6MWT was performed according to the American Thoracic Society standards under the direction of a well-trained respiratory therapist at a 30 m indoor walking course [ 18 ]. Patients were encouraged by the instructor every minute and were allowed to rest or quit the test at any point. We measured the total distance and peripheral saturation with the portable oxygen meter. The patients’ body compositions were estimated indirectly using the BIA from a supine position (InBody S10, InBody, Co. Ltd., Seoul, Korea).
Diaphragm function was assessed using ultrasonography (LOGIQ E9, GE Healthcare; Chicago, IL, USA) obtained from both supine and sitting positions. It is generally accepted that there are positional differences in diaphragm contractility. The effects of gravitational loading on the diaphragm length-tension and body position-mediated changes in intra-abdominal pressure may explain the differences found. Not only that there is also a difference in the excursion between right and left. The excursion of the right diaphragm shows a lower value than that of the left diaphragm because the liver in the abdominal cavity restricts the movement of the right diaphragm. We also measured the diaphragm function in two positions based on this information. The supine position involved lying on the back or with the face upward while the sitting position was semi-seated (45–60 degrees). Both M-mode and B-mode imaging were used to evaluate diaphragmatic excursion and thickness, respectively. The mid-clavicular line and the liver were used as anatomical landmarks on the right side and the spleen on the left side to visualize the diaphragm in the M-mode. B-mode ultrasonography was used to measure the diaphragmatic thickness at the bilateral zone of apposition [ 19 ]. The diaphragm thickness was measured during quiet spontaneous breathing without peak inspiratory or expiratory maneuvers. The diaphragmatic thickness fraction was calculated as the difference between thickness at the end of inspiration and thickness at the end of expiration divided by thickness at the end of expiration x 100. The diaphragmatic excursion was measured as follows. The highest position of the diaphragm movement taken by the M-mode was considered to be the end-expiratory phase, whereas the lowest position was considered as the end-inspiratory phase.
The dyspnea scale used St. George's Respiratory Questionnaire (SGRQ) and the modified Medical Research Council scale (mMRC scale). The SGRQ is a self-administered questionnaire with 76 items [ 20 ]. This can identify the patient’s symptoms and the activities of daily life. mMRC scale is most commonly used in the assessment of dyspnea in chronic respiratory diseases and is a very useful and unrecognized dyspnea scale [ 21 ].
Statistical analysis
The data were analyzed using IBM SPSS (version 27.0; Chicago, IL, USA). The level of significance was set at p < 0.05. Descriptive statistics, including numbers, percentages, means, and standard deviations, were used to summarize each variable (demographics, PFTs, 6MWT, and diaphragmatic ultrasound results). The results were analyzed by independent t-test, cross-analysis, and frequency analysis. The correlation between the variables was analyzed by Pearson’s Correlation Coefficient, which confirmed the linear relationship between two variables using a scatterplot. The cut-off value was calculated using the receiver operating characteristic (ROC) curve analysis. The reference plane was 0.5 or more in the ROC curve, and the p -value < 0.05; hence, this result was adopted. Consequently, the cut-off value was confirmed when sensitivity and specificity were plotted in a line chart, which is the point where the two graphs meet.
Ethics statement
We certify that all applicable institutional and governmental regulations concerning the ethical use of human volunteers were followed throughout this study. The study procedures were reviewed and approved by our Pusan National University Yangsan Hospital Institutional Review Board [IRB No. 05–2020-217].
FEV1 and diaphragm function
We assessed whether diaphragm function was associated with FEV1 (Fig. 1 ). In the total group analysis, both diaphragmatic excursion and thickness were associated with FEV1. However, the diaphragmatic excursion was more associated with FEV1 than thickness. Diaphragmatic excursion during forced breathing and in the supine position had a greater association with FEV1 than breathing at rest and in the sitting position. Additionally, when comparing the right and left under the same conditions, the right was more significant during forced breathing and in the supine position ( r = 0.370, p = 0.004,). Moreover, diaphragmatic thickness at right end-expiration was associated with FEV1. In summary, right ( r = 0.370, p = 0.004) and left ( r = 0.257, p = 0.048) diaphragmatic excursion during forced breathing in the supine position and diaphragmatic thickness at right end-expiration ( r = 0.310, p = 0.016) were significantly associated with FEV1.
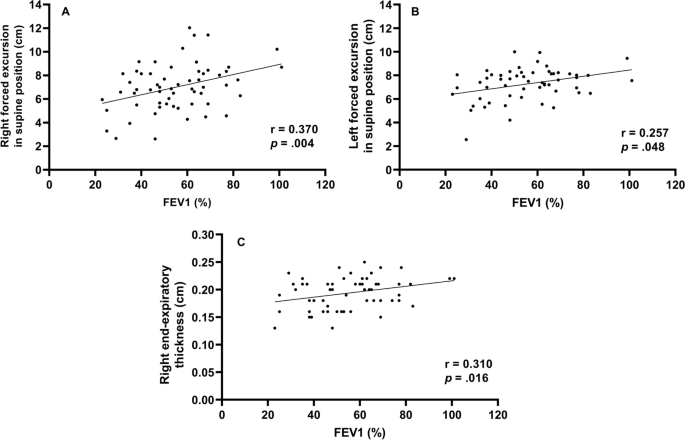
Correlation between forced expiratory volume in 1 s and diaphragm function Right forced excursion, and left forced excursion in the supine position and right end-expiratory thickness were correlated to forced expiratory volume in 1 s
Diaphragmatic function and BMI (body mass index)
To evaluate the function of the diaphragm muscle [ 22 ], the diaphragmatic excursion was measured at rest and during forced expiration (Supplement Table 1 ). In 60 patients, diaphragmatic excursion at rest in the supine position was 3.5 cm ± 1.2 on the right side and 3.5 cm ± 1.2 on the left side. During forced breathing, diaphragmatic excursion in the supine position was 6.9 cm ± 2.0 on the right side and 7.6 cm ± 1.6 on the left side. The total percent body fat was 24.2% ± 6.9. Segmental lean mass analysis was performed by direct segmental multi-frequency BIA. The lean mass was 90.5% ± 9.7 on the right arm, 88.1% ± 9.2 on the left arm, 94.5% ± 5.8 on the trunk, 95.7% ± 131.3 on the right leg, and 9.51% ± 8.8 on the left leg.
Cutoff value-associated characteristics
The ROC curve analysis of the diaphragm function variables was performed to identify the cutoff value for differentiating between FEV1 ≥ 50% and < groups. The cutoff value was ≤ 6.7 cm on the right diaphragmatic excursion at forced breathing with an area under the curve of 0.5 or more and p -value was 0.043. Right diaphragmatic excursion during forced breathing was less than the cut-off value of 6.7 cm for 26 patients and ≥ 6.7 cm for 43 patients (Table 1 ). There were no differences in age, sex, or smoking history between the two groups. The dyspnea scales such as mMRC, SGRQ, and GOLD were not significantly different between both groups. There were no differences in body mass index, percent body fat, or lean mass of the right or left legs between the groups. However, among the pulmonary function indicators, there were significant differences between the two groups. Specifically, FEV1, FVC, and MIP were significantly different (< 6.7 cm group vs. ≥ 6.7 cm group, FEV1: 49.2% ± 16.2 vs. 59.5% ± 17.2, p = 0.021; FVC: 76.2% ± 19.1 vs. 86.0% ± 15.5, p = 0.032; MIP: 67.4 cm H 2 O ± 25.1 vs. 86.5 cm H 2 O ± 28.7, p = 0.010). Concerning the 6MWT, there was a significant difference in SpO2 before 6MWT and the number of interruptions (SpO2 before 6MWT: 94.1% ± 2.7 vs. 95.3% ± 1.6, p = 0.038; number of interruptions: 4 [15.4%] vs. 0 [0%], p = 0.018). The left diaphragmatic excursion during forced breathing was also different between the two groups (6.8 cm ± 1.5 vs. 7.6 cm ± 1.3, p = 0.022) as well as the diaphragmatic thickness during right end-inspiration (0.3 cm ± 0.1 vs. 0.4 cm ± 0.1, p = 0.006). In addition, the ROC ≥ 6.7 cm group left diaphragmatic excursion was also measured with a value greater than that of the ROC < 6.7 cm group.
Subgroup characteristics according to FEV1
To identify the clinical significance of diaphragm function with the relationship between lung function, and COPD severity, the two groups classified as a right diaphragmatic excursion at 6.7 cm of forced breathing were further divided into groups based on FEV1 (< 50% or ≥ 50%) (Table 2 ). There were significant differences in age (65.0 ± 7.8 years vs. 72.7 ± 6.2 years, p = 0.011), the GOLD score ( p < 0.001), FEV1/FVC (40.1% ± 14.7 vs. 55.%4 ± 11.4, p = 0.007), peak expiratory flow rate (183.3 L/min ± 80.4 vs. 275.8 L/min ± 113.8, p = 0.027), SpO2 after the 6MWT (85.9% ± 6.5 vs. 91.5% ± 2.2, p = 0.011), and left diaphragmatic excursion during forced breathing (6.2 cm ± 1.6 vs. 7.4 cm ± 1.0, p = 0.038).
When the group with the right diaphragmatic excursion ≥ 6.7 cm was further divided into subgroups according to FEV1 (< 50% or ≥ 50%) and analyzed, mMRC, GOLD score, FEV1/FVC, MIP, peak expiratory flow rate, 6MWT, SpO2 before and after the 6MWT, and right diaphragmatic thickness at end-expiration subgroups were significantly different between the two groups.
This study contains the following: 1) evidence that FEV1 is significantly correlated with diaphragm movement, 2) cutoff values for diaphragm movement in patients with COPD, and 3) evidence to support the claim that the function of the diaphragm should be considered when interpreting the patient’s condition based on their FEV1.
First, FEV1 was significantly correlated with diaphragm movement. Studies on the relationship between the diaphragm and pulmonary function in patients with COPD are ongoing and have consistently reported that the severity of COPD and diaphragm function are closely related. Some previous studies have evaluated the direct relationship between FEV1 and diaphragm function [ 23 , 24 ].
The results of this study is also consistent with those of previous studies showing that diaphragm movement and FEV1 are related. However, beyond the findings of previous results [ 23 ], in our study, diaphragmatic excursion and thickness were found to be more correlated to FEV1 on the right side than on the left side.
Like the previous study that the thickness of the diaphragm is related to the ventilator weaning mechanical ventilation [ 9 , 10 ], this result has confirmed that the right diaphragm thickness was significantly related not only to the weaning of the ventilator and the prognosis of the patient but also to FEV1.
Second, we provided a cutoff value for a right diaphragmatic forced excursion in patients with COPD. Although there are studies that have presented a reference [ 23 ] value for healthy persons, the significant contribution of this study is the proposed reference value for patients with COPD.
We analyzed the correlation using Pearson’s correlation coefficient and confirmed the factors of diaphragmatic function-related components side (right, left), thickness, and excursion that were most-related to FEV1. Among them, Rt. forced excursion (supine), Lt. forced excursion (supine) and Rt. end-expiratory thickness showed meaningful p -value in association with FEV1. In addition, these three factors were analyzed in the linear relationship with the scattered plot and showed a proportional relationship between FEV1. Finally, when all factors related to the diaphragmatic function were analyzed, the right forced excursion was statistically determined as the most meaningful factor in relation to FEV1. We also obtained the cut-off value of 6.7 cm through the ROC curve.
The range in diaphragmatic excursion values varies considerably depending on the patient’s condition. A previous study has suggested normal values based on sex and the side of the diaphragm using healthy individuals. When breathing deeply, the right diaphragmatic excursion was 7 cm ± 1.1 in men and 5.7 cm ± 1 in women ( p < 0.001) and the left diaphragmatic excursion were 7.5 cm ± 0.9 and 6.4 cm ± 1 in men and women, respectively ( p < 0.01) [ 23 ]. In our study, we also assessed excursion during deep breathing to provide a cut-off value for patients with COPD.
When analyzed by dividing them into two groups based on a cut-off value, the following evaluation factors showed significant differences ( p < 0.05): FEV1, FVC, MIP, left forced excursion, right diaphragmatic thickness during end-inspiration, 6MWT, the SpO2 before and after 6MWT, and interruption of the 6MWT.
These factors can be broadly divided into PFT-related and performance-related factors. As mentioned above, PFT-related factors such as MIP, left diaphragmatic forced excursion and right diaphragmatic thickness during end-inspiration were lower in the < 6.7 cm group. Moreover, the SpO2 level before the 6MWT was lower in the < 6.7 cm group, the overall 6MWT was shorter, and there were many interruptions in the 6MWT. These factors might reflect activity as a performance evaluation factor. Although generalizability is limited given the few patients and the fact that all the participants were outpatients who could walk; these results may reflect an actual patient’s status. However, these findings are intended for patients who can walk, suggesting that the cut-off value of 6.7 cm may be reliable in this population.
Finally, results concerning the degree of pulmonary function and correlations with the diaphragmatic movement were noteworthy. The two groups were analyzed based on the right diaphragmatic forced excursion (6.7 cm) and divided into subgroups based on FEV1 (< 50% vs. ≥ 50%). As a result, in the group that had maintained diaphragm function (≥ 6.7 cm), the MIP, portable peak flow meter, 6MWT, SpO2 before and after the 6MWT, and right diaphragmatic thickness at end-expiration were different between the two FEV1 groups. In summary, the difference between the two FEV1 groups was large when diaphragm function was maintained; when it was not maintained, there were no differences between the two FEV1 groups. Therefore, even in patients who maintained their FEV1 > 50%, when diaphragm function deteriorated, the patient’s 6MWT, SpO2 before and after the 6MWT were less predictable (they either deteriorated or were maintained). The patients whose FEV1 decreased < 50%, if the diaphragm function was maintained, the 6MWT could be better than that in patients with an FEV1 ≥ 50% and a reduced diaphragm function.
In conclusion, when interpreting a patient’s condition based on FEV1, it is important to assess diaphragm function, since the effect of the FEV1 value on the patient depends on how well the diaphragm function has been maintained.
In this study, when the diaphragm function was maintained, there were significant differences in MIP, peak expiratory flow rate, 6MWT, SpO2 before and after the 6MWT, and right diaphragmatic thickness at end-expiration according to FEV1 in patients with COPD. Even if the diaphragm function was not maintained, because there are still differences in the FEV1, it may be beneficial to consider diaphragmatic function measured by right diaphragm excursion as an additional indicator of function beyond the FEV1. Therefore, it can be clinically helpful to check whether the diaphragm is functioning properly when determining a patient’s condition based on FEV1.
Availability of data and materials
The datasets used and/or analysed during the current study are available from the corresponding author on reasonable request.
Abbreviations
Chronic obstructive pulmonary disease
Pulmonary function test
- 6-minute walk test
Forced expiratory volume in the first second
Maximal inspiratory pressure
International Classification of Diseases 11TH
Forced vital capacity
Global Initiative for Chronic Obstructive Lung Disease
Computed tomography
Maximal expiratory pressure
Bioelectrical impedance analysis
Modified Medical Research Council
Receiver operating characteristic
Body mass index
St. George's Respiratory Questionnaire
Niedermeyer J. Dyspnea in airway and pulmonary diseases. Internist. 2015;56(8):882–9.
Article CAS Google Scholar
Antoniu SA. Descriptors of dyspnea in obstructive lung diseases. Multidisciplinary respiratory medicine. 2010;5(3):216–9.
Article Google Scholar
Lopez AD, Shibuya K, Rao C, Mathers CD, Hansell AL, Held LS, et al. Chronic obstructive pulmonary disease: current burden and future projections. Eur Respir J. 2006;27(2):397–412.
Decramer M. Respiratory muscles in COPD: regulation of trophical status Verhandelingen. Koninklijke Academie voor Geneeskunde van Belgie. 2001;63(6):577–602 discussion −4.
CAS Google Scholar
Corbellini C, Boussuges A, Villafañe JH, Zocchi L. Diaphragmatic mobility loss in subjects with moderate to very severe COPD may improve after in-patient pulmonary rehabilitation. Respir Care. 2018;63(10):1271–80.
Crimi C, Heffler E, Augelletti T, Campisi R, Noto A, Vancheri C, et al. Utility of ultrasound assessment of diaphragmatic function before and after pulmonary rehabilitation in COPD patients. Int J Chronic Obstruct Pulmon Dis. 2018;13:3131–9.
He L, Zhang W, Zhang J, Cao L, Gong L, Ma J, et al. Diaphragmatic motion studied by M-mode ultrasonography in combined pulmonary fibrosis and emphysema. Lung. 2014;192(4):553–61.
Laghi F, Tobin MJ. Disorders of the respiratory muscles. Am J Respir Crit Care Med. 2003;168(1):10–48.
Goligher EC, Fan E, Herridge MS, Murray A, Vorona S, Brace D, et al. Evolution of diaphragm thickness during mechanical ventilation. Impact Inspiratory Effort Am J Respirat Cri Care Med. 2015;192(9):1080–8.
Goligher EC, Dres M, Fan E, Rubenfeld GD, Scales DC, Herridge MS, et al. Mechanical ventilation-induced diaphragm atrophy strongly impacts clinical outcomes. Am J Respir Crit Care Med. 2018;197(2):204–13.
Boussuges A, Rives S, Finance J, Brégeon F. Assessment of diaphragmatic function by ultrasonography: current approach and perspectives. World J Clin Cases. 2020;8(12):2408–24.
Sarwal A, Walker FO, Cartwright MS. Neuromuscular ultrasound for evaluation of the diaphragm. Muscle Nerve. 2013;47(3):319–29.
Criner G. 6-minute walk testing in COPD: is it reproducible? Eur Respir J. 2011;38(2):244–5.
Hernandes NA, Wouters EF, Meijer K, Annegarn J, Pitta F, Spruit MA. Reproducibility of 6-minute walking test in patients with COPD. Eur Respir J. 2011;38(2):261–7.
Chen H, Liang BM, Tang YJ, Xu ZB, Wang K, Yi Q, et al. Relationship between 6-minute walk test and pulmonary function test in stable chronic obstructive pulmonary disease with different severities. Chin Med J. 2012;125(17):3053–8.
Google Scholar
Minami T, Manzoor K, McCool FD. Assessing diaphragm function in Chest Wall and neuromuscular diseases. Clin Chest Med. 2018;39(2):335–44.
ATS/ERS Statement on Respiratory Muscle Testing. Am J Respir Crit Care Med. 2002;166(4):518–624.
ATS statement: guidelines for the six-minute walk test. Am J Respir Crit Care Med. 2002;166(1):111–7.
Matamis D, Soilemezi E, Tsagourias M, Akoumianaki E, Dimassi S, Boroli F, et al. Sonographic evaluation of the diaphragm in critically ill patients. Technique and clinical applications. Intensive Care Med. 2013;39(5):801–10.
Jones PW, Quirk FH, Baveystock CM. The St George's respiratory questionnaire. Respir Med. 1991;85 Suppl B(25-31):discussion 3-7.
Launois C, Barbe C, Bertin E, Nardi J, Perotin JM, Dury S, et al. The modified Medical Research Council scale for the assessment of dyspnea in daily living in obesity: a pilot study. BMC pulmonary medicine. 2012;12:61.
Dhungana A, Khilnani G, Hadda V, Guleria R. Reproducibility of diaphragm thickness measurements by ultrasonography in patients on mechanical ventilation. World J Critical Care Med. 2017;6(4):185–9.
Boussuges A, Gole Y, Blanc P. Diaphragmatic motion studied by m-mode ultrasonography: methods, reproducibility, and normal values. Chest. 2009;135(2):391–400.
Rocha FR, Brüggemann AK, Francisco DS, Medeiros CS, Rosal D, Paulin E. Diaphragmatic mobility: relationship with lung function, respiratory muscle strength, dyspnea, and physical activity in daily life in patients with COPD. Jornal brasileiro de pneumologia : publicacao oficial da Sociedade Brasileira de Pneumologia e Tisilogia. 2017;43(1):32–7.
Download references
Acknowledgements
Abstract has been published/presented in the Korean tuberculosis and respiratory society, the Korean tuberculosis and respiratory society fall academic presentation | 129 volume 0342 ~ 343, total 2 PAGES, 2021
https://journal.kstudy.com/ISS_Detail.asp?key=3921544&tname=kiss2002&code=YqldZWtoSqVtJTNEOTEnMSUmN/B%20Z%20xLJTNEVHJpZSUmNbNj2bRU4XB/JTNEMA ==
This study was supported by the Research Institute for Convergence of Biomedical Science and Technology (30–2020-003), Pusan National University Yangsan Hospital. The funding body played no role in the design of the study and collection, analysis, and interpretation of data and in writing the manuscript.
Author information
Taehwa Kim and Sungchul Huh contributed equally to this work.
Authors and Affiliations
Division of Respiratory, Allergy and Critical Care Medicine, Department of Internal Medicine, Pusan National University Yangsan Hospital and Pusan National University School of Medicine, Geumo-ro 20, Beomeo-ri, Yangsan-si, Gyeongsangnam-do, 50612, Republic of Korea
Taehwa Kim, Jae Heun Chung, Yun Seong Kim & Seung Eun Lee
BioMedical Research Institute for Convergence of Biomedical Science and Technology, Pusan National University Yangsan Hospital, Yangsan, South Korea
Department of Rehabilitation Medicine, Rehabilitation Hospital, Pusan National University Yangsan, Yangsan, South Korea
Sungchul Huh & Ra Yu Yun
Pusan National University School of Medicine, Yangsan, South Korea
College of Nursing, Pusan National University, Pusan National University Yangsan Hospital, Yangsan, South Korea
You can also search for this author in PubMed Google Scholar
Contributions
Conceptualization: TK, SEL. Data acquisition and analysis: TK, OP, RYY, SH, JHC, SEL. Data interpretation: TK, RYY, SH, JHC, SEL. Validation: TK, JHC. Writing – original draft: SH, TK. Writing – review: SEL, JHC, YSK. The author(s) read and approved the final manuscript.
Corresponding author
Correspondence to Seung Eun Lee .
Ethics declarations
Ethics approval and consent to participate.
The authors are accountable for all aspects of the work in ensuring that questions related to the accuracy or integrity of any part of the work are appropriately investigated and resolved. The study was conducted in accordance with the Declaration of Helsinki (as revised in 2013) [ 17 ]. The study was approved by Pusan National University Yangsan Hospital (PNUYH) Institutional Review Board (IRB No. 05–2020-217) and individual consent for this retrospective analysis was waived.
Consent for publication
Not applicable.
Competing interests
The authors have no conflicts of interest or funding sources to declare.
Additional information
Publisher’s note.
Springer Nature remains neutral with regard to jurisdictional claims in published maps and institutional affiliations.
Supplementary Information
Additional file 1., rights and permissions.
Open Access This article is licensed under a Creative Commons Attribution 4.0 International License, which permits use, sharing, adaptation, distribution and reproduction in any medium or format, as long as you give appropriate credit to the original author(s) and the source, provide a link to the Creative Commons licence, and indicate if changes were made. The images or other third party material in this article are included in the article's Creative Commons licence, unless indicated otherwise in a credit line to the material. If material is not included in the article's Creative Commons licence and your intended use is not permitted by statutory regulation or exceeds the permitted use, you will need to obtain permission directly from the copyright holder. To view a copy of this licence, visit http://creativecommons.org/licenses/by/4.0/ . The Creative Commons Public Domain Dedication waiver ( http://creativecommons.org/publicdomain/zero/1.0/ ) applies to the data made available in this article, unless otherwise stated in a credit line to the data.
Reprints and permissions
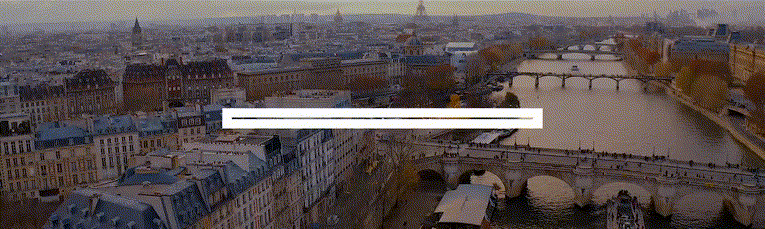
About this article
Cite this article.
Kim, T., Huh, S., Chung, J.H. et al. Clinical values of diaphragmatic movement in patients with chronic obstructive pulmonary disease. BMC Pulm Med 23 , 33 (2023). https://doi.org/10.1186/s12890-022-02220-7
Download citation
Received : 25 April 2022
Accepted : 02 November 2022
Published : 27 January 2023
DOI : https://doi.org/10.1186/s12890-022-02220-7
Share this article
Anyone you share the following link with will be able to read this content:
Sorry, a shareable link is not currently available for this article.
Provided by the Springer Nature SharedIt content-sharing initiative
- Cut-off value
BMC Pulmonary Medicine
ISSN: 1471-2466
- Submission enquiries: [email protected]
- General enquiries: [email protected]
Learn how UpToDate can help you.
Select the option that best describes you
- Medical Professional
- Resident, Fellow, or Student
- Hospital or Institution
- Group Practice
- Find in topic
RELATED TOPICS
INTRODUCTION
Diaphragmatic injury is uncommon, representing much less than 1 percent of all traumatic injuries [ 1,2 ]. The diaphragm is usually injured in association with other thoracic and abdominal organs. Although diaphragmatic injury can be obvious (eg, herniation of abdominal contents on chest radiograph), the injury may be subtle, and imaging studies can be nondiagnostic. A high index of suspicion needs to be maintained because delayed diagnosis is associated with an increased risk for herniation and strangulation of abdominal organs, which can be life-threatening. For patients in whom the diagnosis is uncertain, diagnostic laparoscopy, thoracoscopy, or open surgical exploration may be needed to establish the diagnosis. When identified, diaphragm injury is repaired with open surgical or minimally invasive techniques, the choice and timing of which depends upon the presence of associated injuries and the overall condition of the patient.
This topic will discuss the recognition and surgical management of blunt and penetrating injury to the diaphragm. Injuries to associated thoracic and abdominal organs are discussed in separate topic reviews. The general approach to blunt and penetrating chest and abdominal trauma is also discussed elsewhere. (See "Initial evaluation and management of blunt thoracic trauma in adults" and "Initial evaluation and management of penetrating thoracic trauma in adults" and "Blunt abdominal trauma in adults: Initial evaluation and management" .)
ANATOMY OF THE DIAPHRAGM
The diaphragm (dia: across, phragm: fence) is the musculotendinous boundary between the negative-pressure thoracic cavity and positive-pressure abdominal cavity. The diaphragm plays a significant role in respiratory mechanics, and injury to the diaphragm impairs ventilation and oxygen delivery. The normal mechanics of respiration are discussed elsewhere. (See "Chest wall diseases and restrictive physiology", section on 'Normal structure and function' .)
The diaphragm is dome-shaped and attaches to the chest and abdominal walls circumferentially ( figure 1 ). The liver, spleen, transverse colon, stomach, pancreas, adrenal glands, and kidneys contact the undersurface of the diaphragm. Thoracoabdominal structures, including the aorta, inferior vena cava, thoracic duct, esophagus, vagus nerves, and phrenic nerves, traverse the diaphragm through three major apertures (ie, aortic, caval, esophageal) ( figure 2 ).
The diaphragm is composed of two muscle groups, costal and crural, which are compositionally and functionally distinct. Both groups are innervated by the phrenic nerves ( figure 3 and figure 4 ). The costal muscle group that forms the diaphragmatic leaflets is thin, and contraction of its fibers flattens the diaphragm and lowers the ribs. The crural muscle groups are thicker but contribute minimally to the displacement of the diaphragm. The median arcuate ligament anterior to the aortic hiatus is formed by the continuation of the medial tendinous margins of the crura.
- Open access
- Published: 22 August 2024
Ability of parasternal intercostal muscle thickening fraction to predict reintubation in surgical patients with sepsis
- Mina Adolf Helmy 1 ,
- Ahmed Hasanin 1 ,
- Lydia Magdy Milad 1 ,
- Maha Mostafa 1 ,
- Walid I Hamimy 1 ,
- Rimon S Muhareb 1 &
- Heba Raafat 1
BMC Anesthesiology volume 24 , Article number: 294 ( 2024 ) Cite this article
243 Accesses
Metrics details
We aimed to evaluate the ability of the parasternal intercostal (PIC) thickening fraction during spontaneous breathing trial (SBT) to predict the need for reintubation within 48 h after extubation in surgical patients with sepsis.
This prospective observational study included adult patients with sepsis who were mechanically ventilated and indicated for SBT. Ultrasound measurements of the PIC thickening fraction and diaphragmatic excursion (DE) were recorded 15 min after the start of the SBT. After extubation, the patients were followed up for 48 h for the need for reintubation. The study outcomes were the ability of the PIC thickening fraction (primary outcome) and DE to predict reintubation within 48 h of extubation using area under receiver characteristic curve (AUC) analysis. The accuracy of the model including the findings of right PIC thickening fraction and right DE was also assessed using the current study cut-off values. Multivariate analysis was performed to identify independent risk factors for reintubation.
We analyzed data from 49 patients who underwent successful SBT, and 10/49 (20%) required reintubation. The AUCs (95% confidence interval [CI]) for the ability of right and left side PIC thickening fraction to predict reintubation were 0.97 (0.88–1.00) and 0.96 (0.86–1.00), respectively; at a cutoff value of 6.5–8.3%, the PIC thickening fraction had a negative predictive value of 100%. The AUCs for the PIC thickening fraction and DE were comparable; and both measures were independent risk factors for reintubation. The AUC (95% CI) of the model including the right PIC thickening fraction > 6.5% and right DE ≤ 18 mm to predict reintubation was 0.99 (0.92–1.00), with a positive predictive value of 100% when both sonographic findings are positive and negative predictive value of 100% when both sonographic findings are negative.
Conclusions
Among surgical patients with sepsis, PIC thickening fraction evaluated during the SBT is an independent risk factor for reintubation. The PIC thickening fraction has an excellent predictive value for reintubation. A PIC thickening fraction of ≤ 6.5–8.3% can exclude reintubation, with a negative predictive value of 100%. Furthermore, a combination of high PIC and low DE can also indicate a high risk of reintubation. However, larger studies that include different populations are required to replicate our findings and validate the cutoff values.
Peer Review reports
Introduction
Nearly one-third of critically ill patients require mechanical ventilation [ 1 ]. Prolonged mechanical ventilation is associated with poor patient outcomes and increases the burden on healthcare systems, particularly in the post pandemic era [ 2 ]. Conversely, premature extubation usually results in reintubation, which is an independent risk factor for mortality [ 3 , 4 ].
The optimal point for discontinuing invasive mechanical ventilation should include parameters that prevent both premature extubation and unnecessary prolonged ventilation. The most widely agreed method to reach this point is assessment of the ability of the patient to breathe with no or minimal respiratory support for 30–120 min (spontaneous breathing trial [SBT]) [ 5 ]. The success of SBT is usually evaluated on the basis of major respiratory and cardiovascular signs, such as respiratory rate, gas exchange, and hemodynamic parameters. However, there is an increased interest in the evaluation of respiratory muscular dysfunction as an important and unreplaceable measure of weaning eligibility [ 6 ]. The evaluation of diaphragmatic function has gained the highest attention for its feasibility at the bedside using point-of-care ultrasound [ 6 ]. More recently, the parasternal intercostal (PIC) muscle thickening fraction showed good performance in the evaluation of patients receiving mechanical ventilation during weaning [ 7 , 8 ]. Evaluation of the PIC has some advantages for being close to the skin and not affected by gaseous distension; these characteristics increased the interest in its use for respiratory muscle assessment because it is much easier than diaphragmatic examination with nearly the same accuracy [ 7 , 8 ].
The PIC thickening fraction showed good performance in determining SBT failure [ 7 , 9 ]. However, there are two types of failed SBT: failure to complete the SBT and failure of extubation (reintubation). Previous studies have evaluated the accuracy of PIC in detecting failed SBT, but no studies have evaluated its accuracy for reintubation, which is more serious than failed SBT; thus, detecting reintubation warrants separate investigation. Furthermore, the PIC thickening fraction was not previously evaluated in patients with sepsis. Sepsis can produce several forms of neuromyopathy in different muscles, including the diaphragm [ 10 , 11 ]; reduces the blood supply of respiratory muscles; and damages contractile proteins [ 11 , 12 ], aggravating the effect of mechanical ventilation on respiratory muscles [ 13 , 14 ]. The preferential effect of sepsis on diaphragmatic function indicates that this group of patients requires a separate evaluation of respiratory muscles compared with other critically ill patients [ 12 ].
This study aimed to evaluate the ability of the PIC thickening fraction to predict reintubation in surgical patients with sepsis. The secondary aim was to compare the PIC thickening fraction with the diaphragmatic excursion (DE) as predictors of weaning outcomes.
Patients and methods
This prospective observational study was conducted in the surgical intensive care unit at Cairo University Hospital between September 2022 and July 2023 after institutional research ethics committee approval (Cairo University’s Research Ethics Committee approval no. MD-167-2022). Written informed consent was obtained from the patient’s next of kin.
We consecutively included adult (> 18 years) surgical patients with sepsis who were mechanically ventilated for ≥ 24 h. The diagnosis and management of the patients were based on the latest guidelines [ 15 ].
Patients with diaphragmatic paralysis and neuromuscular diseases and pregnant women were excluded.
The attending intensivist assessed the patients for weaning eligibility, which included PaO 2 > 60 mmHg at positive end expiratory pressure ≤ 8 cmH 2 O, appropriate pH and PaCO 2 , and hemodynamic stability (or on low-dose vasopressors). All included patients were fully conscious and had a good cough reflex [ 16 ].
Eligible patients underwent SBT for 120 min using a positive end expiratory pressure of 5 cm H 2 O and pressure support of 5 cmH 2 O. The SBT was considered a failure when any of the following was present: respiratory rate > 35 breaths per minute, increased work of breathing, SpO 2 < 90% or PaO 2 < 60 mmHg on FiO 2 > 0.4, or hemodynamic instability (hear rate > 140 beats per minute or > 20% change from baseline and systolic blood pressure > 180 mmHg or > 20% change from baseline) [ 16 ]. Patients who successfully passed the SBT were extubated and were observed for the next 48 h for reintubation. A simple oxygen mask was used to maintain SpO 2 > 92%. Failed weaning was defined as either failed SBT or the need for reintubation within 48 h after extubation.
An experienced intensivist (LM) who had conducted > 150 previous similar examinations performed ultrasound measurements. Ultrasound examinations were performed using a Versana Essential device (GE Medical Systems Co., Ltd., China) 15 min after initiating the SBT in the semi-sitting position.
Ultrasound assessment of PIC thickening fraction
A high-frequency linear transducer (L6-12-RS, 4–16 MHz) was placed vertically at the second intercostal space, 3 cm lateral to the sternal border. Inspiratory and expiratory muscle thickness was measured using the M-mode. The PIC thickening fraction was calculated as (inspiratory muscle thickness − expiratory muscle thickness/expiratory muscle thickness) ×100% (Fig. 1 ).

Ultrasound assessment of respiratory muscle. A : Transducer placement for assessment of parasternal intercostal thickening fraction. B : Inspiratory (yellow line 1) and expiratory (yellow line 2) intercoastal muscle thickness were measured using the M-mode. C : Transducer placement for assessment of diaphragmatic excursion. D : The amplitude of diaphragmatic muscle (yellow arrow) excursion between inspiration and expiation (yellow line 3) was then measured using the M-mode. IC: intercostal muscle
Ultrasound assessment of DE
A low-frequency curved transducer (4 C-RS, 2–5 MHz) was placed horizontally at one of the lower intercostal spaces on the right anterior axillary line and left midaxillary line for the right and left sides, respectively. The patient was then asked to take a maximum breath, after which the amplitude of excursion between inspiration and expiration was measured using the M-mode (Fig. 1 ).
The mean PIC thickening fraction and DE were calculated as the average of the right and left side measurements.
Clinicians in charge of patient care were blinded to ultrasound measurements.
The primary outcome was the ability of the PIC thickening fraction to predict reintubation after successful SBT. The secondary outcomes included the accuracy of PIC thickening fraction and DE in predicting successful weaning. The cutoff values obtained from the area under the curve (AUC) analysis for the right PIC thickening fraction and right DE were used to categorize the patients into either positive or negative risk for reintubation. Positive risk for reintubation was considered when the PIC thickening fraction was higher than the obtained cutoff value and/or DE was lower than the cutoff value, and vice versa for negative risk for reintubation. Each patient had either two positive sonographic findings, one positive sonographic finding, or two negative sonographic findings, and the accuracy of this model was assessed. Patient demographic data, comorbidities, Acute Physiology and Chronic Health Evaluation (APACHE) II score, hemodynamic and respiratory parameters (PaO 2 /FiO 2 ratio PaCO 2 , respiratory rate, and rapid shallow breathing index [RSBI]), cause of mechanical ventilation, days of mechanical ventilation, and source of sepsis were recorded.
Sample size
Sample size calculation was performed using MedCalc version 18 (MedCalc Software bvba, Ostend, Belgium). Assuming an incidence of reintubation of 20%, a minimum sample size of 45 patients (at least nine reintubation cases) was needed to detect an AUC of 0.8 with the null hypothesis set at 0.5 for a study power of 80% and alpha error of 0.05.
Statistical analysis
Patients were divided according to 1- weaning outcome (failed weaning, including reintubation or failed SBT versus successful weaning), 2- the need to reintubation versus successful extubation. The Schapiro–Wilk test was implemented to evaluate data distribution; normally distributed data are reported as mean ± standard deviation and were analyzed using the independent sample t-test, whereas skewed data are reported as median (quartiles) and were analyzed using the Mann-Whitney test. Categorical variables are summarized as counts and percentages and were analyzed using the Chi-squared or Fisher’s exact test as appropriate. The AUC was calculated to assess the ability of PIC and DE to predict weaning outcome and reintubation. Youden’s index was used to determine the best cutoff value. Logistic regression was used to calculate odds ratios and 95% confidence intervals (CIs) for the prediction of failed weaning and reintubation. Multivariate analysis using forward selection method was performed to identify independent predictors of failed weaning and reintubation. The model included age, the APACHE score (failed weaning model only), duration of mechanical ventilation, RSBI, mean DE, and mean PIC thickening fraction. MedCalc version 14 and SPSS (version 26) for Microsoft Windows (Armonk, NY: IBM Corp.) were used for statistical analysis.
Among the 71 patients who fulfilled the weaning criteria, seven patients were excluded. Sixty-four patients were included and were available for final analysis; 15 patients had failed SBT. Forty-nine patients underwent successful SBT, and 10 of 49 (20%) patients were reintubated within 48 h (Fig. 2 ).
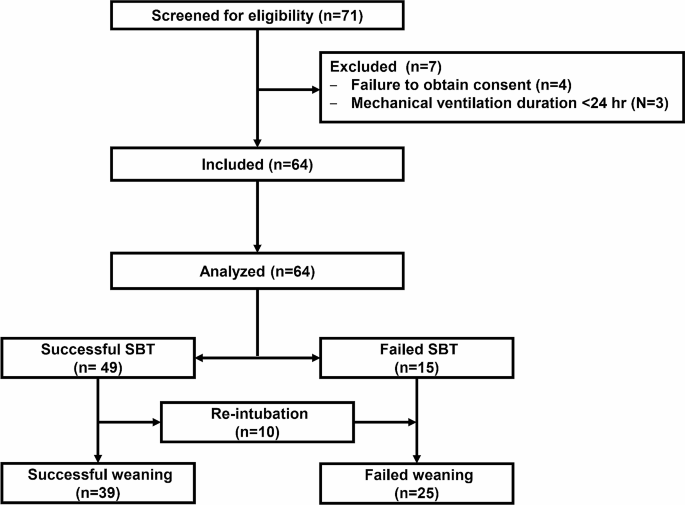
Patients’ enrollment flowchart
Patient demographic, clinical, and laboratory data are presented in Table 1 . Hemodynamic instability and respiratory failure were the main causes of mechanical ventilation (Table 1 ).
The AUC (95% CI) for the ability of the right and left PIC thickening fraction to predict reintubation was 0.97 (0.88–1.00) and 0.96 (0.86–1.00), respectively, and for the prediction of failed weaning, the PIC thickening fraction AUC was 0.98 (0.91–1.00) and 0.97 (0.90–1.00), respectively (Table 2 ).
At a cutoff value of > 6.5–8.3%, the PIC thickening fraction had a negative predictive value of 100% for the prediction of reintubation. The AUCs for the ability of the PIC thickening fraction to predict reintubation and failed weaning were comparable with those for DE (Table 2 ).
The AUC (95% CI) of the model, including right PIC thickening fraction > 6.5% and right DE ≤ 18 mm, for predicting reintubation was 0.99 (0.92–1.00). The presence of one positive sonographic finding had a sensitivity of 100 (69–100)%, specificity of 87 (73–96)%, positive predictive value of 67 (38–88)%, and negative predictive value of 100 (90–100)%. The presence of both positive sonographic findings had a sensitivity of 90 (56–100)%, specificity of 100 (91–100)%, positive predictive value of 100 (66–100)%, and negative predictive value of 98 (87–100)%. Figure 3 presents the proposed algorithm for identifying the risk of reintubation based on right-side sonographic findings.
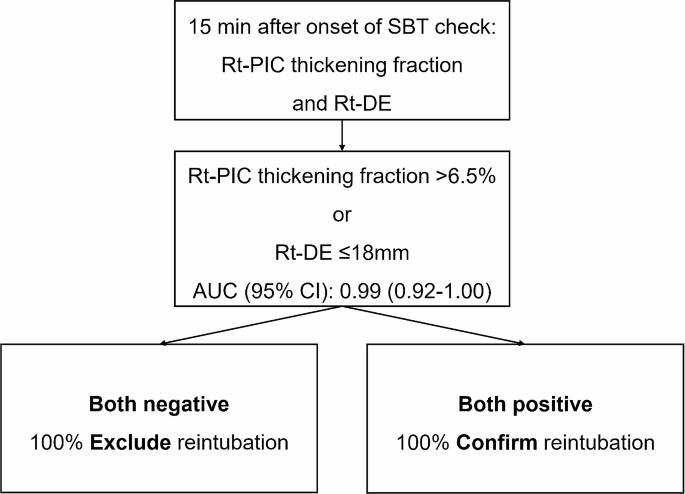
A proposed approach for utilizing the ultrasound assessment of both Rt-PIC thickening fraction and Rt-DE during the SBT in predicting reintubation within 48 h. AUC: area under receiver operating characteristic curve, CI: confidence interval, Rt-DE: right diaphragmatic excursion, Rt-PIC: right parasternal intercostal muscle, SBT: spontaneous breathing trial
Univariate analysis revealed that increased age, duration of mechanical ventilation, respiratory rate, RSBI, PIC thickening fraction, and lower DE were risk factors for reintubation (Supplementary Tables 1 and 2 ).
Multivariate analysis, including the significant parameters from the univariate analysis, revealed that the independent predictors of reintubation and failed weaning were high PIC thickening fraction and low DE (Table 3 ).
Our findings revealed that the PIC thickening fraction could accurately predict both failed weaning and reintubation in patients with sepsis. Furthermore, PIC was an independent risk factor for reintubation. We also found that a combination of impaired diaphragmatic function and increased PIC muscle activity could perfectly predict reintubation, whereas the absence of both findings could predict successful extubation. The role of SBT is to evaluate extubation readiness by examining the balance between ventilatory workload and drive. The intercostal muscles are accessory respiratory muscles that exhibit increased activity proportionate to excessive work of breathing [ 7 ]. This increased activity compensates for the impaired diaphragmatic function [ 7 ] leading to an inverse relationship between the PIC thickening fraction and DE [ 7 , 17 ]. Thus, the PIC thickening fraction can be used as a tool for evaluating current or pending respiratory distress, with the advantage of being easier than diaphragmatic ultrasound indices. Supporting this explanation, Formenti et al. demonstrated that the PIC muscles contribute to the respiratory drive in patients with respiratory distress by impeding the paradoxical inward displacement of the ribs. Formenti et al. concluded that the presence of unphysiological activity of PIC muscles denotes excessive recruitment of accessory inspiratory muscles, which reflects respiratory distress [ 18 ]. In another study, the same authors found a significant decrease in the thickness of both the PIC muscles and diaphragm in the early stage of intensive care unit stay [ 19 ].
Previous data on the use of the PIC thickening fraction support our findings. Dres et al. found that PIC thickening could predict SBT failure [ 7 ], even in patients with normal diaphragmatic thickness [ 9 ]. PIC thickening also predicts noninvasive ventilation failure in patients with COVID-19 [ 20 ]. More recently, PIC thickening was found to be a good tool for risk stratification in the early phase of hospital admission in patients with COVID-19 [ 8 ]. This study provides new insights into the use of intercostal muscle ultrasound for being the first study to evaluate the ability of PIC thickening to predict reintubation. Reintubation is associated with critical airway and respiratory complications, making its prediction more crucial than a failed SBT [ 21 , 22 , 23 ]. Moreover, our study strictly included patients with sepsis who are at high risk of preferential respiratory muscle dysfunction due to reduced blood supply as well as direct damage to contractile proteins [ 12 , 24 ].
Interestingly, the cutoff value in our study for the PIC thickening fraction (≈ 8%) was close to that of previous studies that evaluated the same measurements in other populations. Dres et al. reported that a PIC thickening fraction of > 9.5% during SBT could predict failure [ 7 ]. Helmy et al. found that a PIC thickening fraction of > 9% predicted noninvasive ventilation failure in patients with COVID-19 [ 20 ]; the same authors found that a PIC thickening of > 8.3% on hospital admission predicted the need for ventilatory support in patients with COVID-19 [ 8 ].
In this study, we assessed the ability to combine the PIC thickening fraction and DE to predict reintubation. We report that if none of the parameters were positive for reintubation, the occurrence of reintubation with 100% accuracy will be excluded, whereas if both parameters were positive for reintubation, the occurrence of reintubation with 100% accuracy will be confirmed. Furthermore, this model demonstrated excellent accuracy with a near-perfect AUC (AUC = 0.99). The cutoff values were obtained from the right-side intercostal muscle and hemidiaphragm. We selected the assessment of the right side for the proposed algorithm for several reasons. First, assessment of the right hemidiaphragm is easier than that of the left hemidiaphragm because of the presence of the liver, which provides a wider acoustic window than the spleen on the left side. Second, according to a recent expert consensus, unilateral assessment of the right hemidiaphragm is acceptable and can replace assessment of both sides [ 25 ]. Incorporating several variables into the assessment of respiratory status usually aims to achieve higher accuracy than the use of single variables [ 26 ]. Several combinations and ratios are commonly used in daily practice, such as the PaO 2 /FiO 2 ratio and RSBI. More recently, the incorporation of respiratory muscle ultrasound-derived variables into classic parameters showed good results, such as the diaphragmatic RSBI [ 27 ]. According to our results, we found that the combination of DE and PIC can provide additional insights into respiratory evaluation during weaning.
Reintubation is a major adverse event that is independently responsible for poor outcomes in critically ill patients [ 22 , 23 ]. Extubation failure is associated with a 5-fold increase in mortality [ 28 ]. Thus, predicting patients who are likely to fail is a hot topic in daily critical care practice. Early detection of high-risk patients could prevent premature extubation and enhance investigative and therapeutic measures for the cause of failure. High-risk patients for reintubation might also benefit from receiving noninvasive respiratory support after extubation. The use of noninvasive positive pressure ventilation and/or high-flow nasal oxygen reduced the incidence of failure after extubation [ 29 , 30 , 31 ]. However, these interventions are not devoid of disadvantages, such as cost, patient discomfort, and gastric insufflation; therefore, the use of noninvasive ventilation after weaning is still not routinely implemented in all patients, and it is unclear how to select patients who can benefit from it [ 32 , 33 ]. High-risk patients for reintubation might be an appropriate subgroup that can benefit from prophylactic respiratory support after extubation to prevent reintubation [ 32 , 33 ]. Our findings could help to identify these patients. The COVID-19 pandemic produced an unusual situation with overwhelming need for ventilators and intensive care beds. This crisis highlighted the importance of a rapid and efficient weaning process that provides appropriate extubation decision and avoid unnecessary delay in bed clearance for new admissions.
The use of point-of-care ultrasound is expanding exponentially in critical care medicine [ 34 ] because it is feasible at the bedside, noninvasive, and effective in the examination of several organs in a short period. Point-of-care ultrasound has become a basic skill for acute care physicians [ 35 , 36 ]. The most common and easy ultrasound tool for measuring respiratory function is DE. Our results support the available literature regarding the accuracy of DE in predicting weaning failure and adding PIC thickening fraction, which showed nearly the same predictive values as DE with the advantage of being much easier. Furthermore, we introduced a novel combination of both DE and PIC to rule in and out successful extubation.
Our study has limitations, such as being a single-center observational study confined to a specific population with surgical sepsis. A single operator performed all ultrasound measurements; however, previous studies have shown that ultrasound assessment of respiratory muscle has good reproducibility [ 7 ]. Furthermore, we recorded the respiratory data and ultrasound measurements at a single time point during the SBT; therefore, temporal changes could not be assessed. Future larger studies are needed to confirm our findings in other types of patients and to assess ultrasound measurements at multiple time points during the SBT to identify the optimum timing of measurement and definitive cutoff value for implementation in clinical practice. We did not measure the maximum inspiratory pressure during the SBT; therefore, future studies are needed to assess the relationship between respiratory muscle function and maximum inspiratory pressure and the risk of reintubation.
In conclusion, among surgical patients with sepsis, PIC thickening fraction evaluated during the SBT is an independent risk factor for both weaning failure and reintubation. The PIC thickening fraction has an excellent predictive value for reintubation. A PIC thickening fraction of ≤ 6.5–8.3% can exclude reintubation with 100% accuracy. The combination of high PIC and low DE can also indicate a high risk of reintubation. However, larger studies that include different populations are needed to replicate our findings and validate the cutoff values.
Data availability
The datasets used and/or analyzed during the current study are available from the corresponding author on reasonable request.
Abbreviations
Acute Physiology and Chronic Health Evaluation
Area under receiver operating characteristic curve
Body mass index
Confidence interval
Coronavirus disease
- Diaphragmatic excursion
Mean arterial blood pressure, M-DE: mean diaphragmatic excursion
Mean parasternal intercostal
Mechanical ventilation NPV: negative predictive value
Positive predictive value
Parasternal intercostal muscle
Respiratory rate
Rapid shallow breathing index
- Spontaneous breathing trial
Statistical package for social science
Anzueto A, Frutos F, Brochard L, Stewart TE, Benito S, Epstein SK, et al. Characteristics and outcomes in adult patients receiving mechanical ventilation. JAMA. 2002;287:345–55.
Article PubMed Google Scholar
Zilberberg MD, de Wit M, Pirone JR, Shorr AF. Growth in adult prolonged acute mechanical ventilation: implications for healthcare delivery. Crit Care Med. 2008;36:1451–5.
Torres A, Gatell JM, Aznar E, El-ebiary M, Puig J, Bellacasa DELA, et al. Re-intubation increases the risk of nosocomial pneumonia in patients needing mechanical ventilation. AM J RESPIR CRIT CARE MED. 1995;152:137–41.
Article CAS PubMed Google Scholar
Epstein SK, Ciubotaru RL. Independent effects of etiology of failure and time to reintubation on outcome for patients failing extubation. Am J Respir Crit Care Med. 1998;158:489–93.
McConville JF, Kress JP. Weaning patients from the Ventilator. N Engl J Med. 2012;367:2233–9.
Tuinman PR, Jonkman AH, Dres M, Shi ZH, Goligher EC, Goffi A, et al. Respiratory muscle ultrasonography: methodology, basic and advanced principles and clinical applications in ICU and ED patients — a narrative review. Intensive Care Med. 2020. https://doi.org/10.1007/s00134-019-05892-8 .
Article PubMed PubMed Central Google Scholar
Dres M, Dubé BP, Goligher E, Vorona S, Demiri S, Morawiec E, et al. Usefulness of Parasternal Intercostal Muscle Ultrasound during Weaning from Mechanical Ventilation. Anesthesiology. 2020;132:1114–25.
Helmy MA, Milad LM, Hasanin AM, Mostafa M, Mannaa AH, Youssef MM, et al. Parasternal intercostal thickening at hospital admission: a promising indicator for mechanical ventilation risk in subjects with severe COVID 19. J Clin Monit Comput. 2023. https://doi.org/10.1007/s10877-023-00989-4 .
He G, Han Y, Zhan Y, Yao Y, Zhou H, Zheng X. The combined use of parasternal intercostal muscle thickening fraction and P0.1 for prediction of weaning outcomes. Heart Lung. 2023;62:122–8.
Oliveira TS, Santos AT, Andrade CBV, Silva JD, Blanco N, de Rocha N. Sepsis disrupts mitochondrial function and diaphragm morphology. Front Physiol. 2021;12:704044.
Chen Y, Liu Y, Han M, Zhao S, Tan Y, Hao L, et al. Quantification of diaphragmatic dynamic dysfunction in septic patients by bedside ultrasound. Sci Rep. 2022;12:17336.
Article CAS PubMed PubMed Central Google Scholar
Jung B, Nougaret S, Conseil M, Coisel Y, Futier E, Chanques G, et al. Sepsis is Associated with a preferential diaphragmatic atrophy: a critically ill patient study using Tridimensional Computed Tomography. Anesthesiology. 2014;120:1182–91.
Dres M, Goligher EC, Heunks LMA, Brochard LJ. Critical illness associated diaphragm weakness. Intensive Care Med. 2017;43:1441–52.
Petrof BJ. Diaphragmatic dysfunction in the intensive care unit: Caught in the cross-fire between sepsis and mechanical ventilation. Crit Care. 2013;17.
Opal SM, Rubenfeld GD, Poll T, Van Der, Vincent J, Angus DC. The Third International Consensus definitions for Sepsis and septic shock (Sepsis-3). JAMA. 2016;315:801–10.
Peñuelas Ó, Thille AW, Esteban A. Discontinuation of ventilatory support: new solutions to old dilemmas. Curr Opin Crit Care. 2015;21:74–81.
Schmidt M, Kindler F, Gottfried SB, Raux M, Hug F, Similowski T, et al. Dyspnea and surface inspiratory electromyograms in mechanically ventilated patients. Intensive Care Med. 2013;39:1368–76.
Formenti P, Umbrello M, Dres M, Chiumello D. Ultrasonographic assessment of parasternal intercostal muscles during mechanical ventilation. Ann Intensiv Care. 2020;10.
Paolo F, Valentina DG, Silvia C, Tommaso P, Elena C, Martin D et al. The possible predictive value of muscle ultrasound in the diagnosis of ICUAW in long-term critically ill patients. J Crit Care. 2022;71.
Helmy MA, Hasanin A, Milad LM, Mostafa M, Fathy S. Parasternal intercostal muscle thickening as a predictor of non-invasive ventilation failure in patients with COVID-19. Anaesth Crit Care Pain Med. 2022;41:101063.
Elmer J, Lee S, Rittenberger JC, Dargin J, Winger D, Emlet L. Reintubation in critically ill patients: procedural complications and implications for care. Crit Care. 2015;19:12.
Thille AW, Harrois A, Schortgen F, Brun-Buisson C, Brochard L. Outcomes of extubation failure in medical intensive care unit patients. Crit Care Med. 2011;39:2612–8.
Frutos-Vivar F, Esteban A, Apezteguia C, González M, Arabi Y, Restrepo MI, et al. Outcome of reintubated patients after scheduled extubation. J Crit Care. 2011;26:502–9.
Chen Y, Liu Y, Han M, Zhao S, Tan Y, Hao L, et al. Quantification of diaphragmatic dynamic dysfunction in septic patients by bedside ultrasound. Sci Rep. 2022;12:1–8.
Google Scholar
Haaksma ME, Smit JM, Boussuges A, Demoule A, Dres M, Ferrari G, et al. EXpert consensus on Diaphragm UltraSonography in the critically ill (EXODUS): a Delphi consensus statement on the measurement of diaphragm ultrasound-derived parameters in a critical care setting. Crit Care. 2022;26:1–9.
Article Google Scholar
Vetrugno L, Orso D, Corradi F, Zani G, Spadaro S, Meroi F, et al. Diaphragm ultrasound evaluation during weaning from mechanical ventilation in COVID-19 patients: a pragmatic, cross-section, multicenter study. Respir Res. 2022;23:1–7.
Spadaro S, Grasso S, Mauri T, Dalla Corte F, Alvisi V, Ragazzi R, et al. Can diaphragmatic ultrasonography performed during the T-tube trial predict weaning failure? The role of diaphragmatic rapid shallow breathing index. Crit Care. 2016;20:1–11.
You A, It L. Tracheal reintubation: caused by too much of a good thing ? Respir Care. 2012;57:1687–91.
Hernández G, Paredes I, Moran F, Buj M, Colinas L, Rodríguez ML, et al. Effect of postextubation noninvasive ventilation with active humidification vs high-flow nasal cannula on reintubation in patients at very high risk for extubation failure: a randomized trial. Intensive Care Med. 2022;48:1751–9.
Hernández G, Vaquero C, González P, Subira C, Frutos-Vivar F, Rialp G, et al. Effect of Postextubation High-Flow nasal cannula vs conventional oxygen therapy on Reintubation in Low-Risk patients: a Randomized Clinical Trial. JAMA. 2016;315:1354–61.
Thille AW, Muller G, Gacouin A, Coudroy R, Decavèle M, Sonneville R, et al. Effect of Postextubation High-Flow Nasal Oxygen with Noninvasive Ventilation vs High-Flow nasal oxygen alone on Reintubation among patients at high risk of Extubation failure: a Randomized Clinical Trial. JAMA. 2019;322:1465–75.
Casey JD, Vaughan EM, Lloyd BD, Billas PA, Jackson KE, Hall EJ, et al. Protocolized Postextubation respiratory support to prevent Reintubation: a Randomized Clinical Trial. Am J Respir Crit Care Med. 2021;204:294–302.
Hernández G, Vaquero C, Ortiz R, Colinas L, de Pablo R, Segovia L, et al. Benefit with preventive noninvasive ventilation in subgroups of patients at high-risk for reintubation: a post hoc analysis. J Intensive Care. 2022;10:43.
Vieillard-Baron A, Millington SJ, Sanfilippo F, Chew M, Diaz-Gomez J, McLean A, et al. A decade of progress in critical care echocardiography: a narrative review. Intensive Care Med. 2019;45:770–88.
Robba C, Wong A, Poole D, Al Tayar A, Arntfield RT, Chew MS, et al. Basic ultrasound head-to-toe skills for intensivists in the general and neuro intensive care unit population: consensus and expert recommendations of the European Society of Intensive Care Medicine. Intensive Care Med. 2021;47:1347–67.
Atkinson P, Bowra J, Lambert M, Lamprecht H, Noble V, Jarman B. International Federation for Emergency Medicine point of care ultrasound curriculum. CJEM. 2015;17:161–70.
Download references
Acknowledgements
Not applicable.
The authors declare that no funds, grants, or other support were received during the preparation of this manuscript.
Open access funding provided by The Science, Technology & Innovation Funding Authority (STDF) in cooperation with The Egyptian Knowledge Bank (EKB).
Author information
Authors and affiliations.
Department of Anesthesia and Critical Care Medicine, Faculty of Medicine, Cairo University, Cairo, Egypt
Mina Adolf Helmy, Ahmed Hasanin, Lydia Magdy Milad, Maha Mostafa, Walid I Hamimy, Rimon S Muhareb & Heba Raafat
You can also search for this author in PubMed Google Scholar
Contributions
All authors contributed to the study conception and design. Material preparation, data collection and analysis were performed by [MH, AH, LM, MM, WH, RM, HR]. The first draft of the manuscript was written by [AH, MH, MM] and all authors commented on previous versions of the manuscript. All authors read and approved the final manuscript.
Corresponding author
Correspondence to Ahmed Hasanin .
Ethics declarations
Ethics approval and consent to participate.
This prospective observational study was conducted in Cairo University Hospital after institutional ethics committee approval (MD-167-2022). This study was performed in line with the principles of the Declaration of Helsinki. Written informed consents were obtained from patient’s next-of-kin.
Consent for publication
not applicable.
Conflict of interest
The authors have no relevant financial or non-financial interests to disclose.
Additional information
Publisher’s note.
Springer Nature remains neutral with regard to jurisdictional claims in published maps and institutional affiliations.
Electronic supplementary material
Below is the link to the electronic supplementary material.
Supplementary Material 1
Rights and permissions.
Open Access This article is licensed under a Creative Commons Attribution 4.0 International License, which permits use, sharing, adaptation, distribution and reproduction in any medium or format, as long as you give appropriate credit to the original author(s) and the source, provide a link to the Creative Commons licence, and indicate if changes were made. The images or other third party material in this article are included in the article’s Creative Commons licence, unless indicated otherwise in a credit line to the material. If material is not included in the article’s Creative Commons licence and your intended use is not permitted by statutory regulation or exceeds the permitted use, you will need to obtain permission directly from the copyright holder. To view a copy of this licence, visit http://creativecommons.org/licenses/by/4.0/ . The Creative Commons Public Domain Dedication waiver ( http://creativecommons.org/publicdomain/zero/1.0/ ) applies to the data made available in this article, unless otherwise stated in a credit line to the data.
Reprints and permissions
About this article
Cite this article.
Helmy, M.A., Hasanin, A., Milad, L.M. et al. Ability of parasternal intercostal muscle thickening fraction to predict reintubation in surgical patients with sepsis. BMC Anesthesiol 24 , 294 (2024). https://doi.org/10.1186/s12871-024-02666-8
Download citation
Received : 15 April 2024
Accepted : 31 July 2024
Published : 22 August 2024
DOI : https://doi.org/10.1186/s12871-024-02666-8
Share this article
Anyone you share the following link with will be able to read this content:
Sorry, a shareable link is not currently available for this article.
Provided by the Springer Nature SharedIt content-sharing initiative
- Parasternal thickening
- Mechanical ventilation
- Failed weaning
- Reintubation
BMC Anesthesiology
ISSN: 1471-2253
- General enquiries: [email protected]
Diaphragm: The Relationship between Blood Supply Regulation and Characteristics of the Contractile Function
- Published: 15 September 2020
- Volume 75 , pages 41–49, ( 2020 )
Cite this article
- A. A. Borzykh 1 ,
- O. L. Vinogradova 1 , 2 &
- O. S. Tarasova 1 , 3
249 Accesses
3 Citations
Explore all metrics
The diaphragm is a unique skeletal muscle; it is active throughout the lifetime and, therefore, differs from locomotor muscles in the properties of muscle fibers and the mechanisms of blood supply control. The aim of this review was to survey the structural characteristics of diaphragm muscle tissue, which provide its integral contractile properties; to compare the activity of vascular tone control mechanisms in the diaphragm and locomotor muscles; and to explore their relationship with the regulation of contractile function. The diaphragm differs from the majority of skeletal muscles by a high content of both slow fatigue-resistant muscle fibers of type I and fast fibers of type IIb, which provides endurance and high force-velocity characteristics of the diaphragm. The muscle fibers in the diaphragm are smaller, and the density of capillarization is much higher than in locomotor muscles. Arteries and arterioles that regulate blood supply to the diaphragm capillary bed combine the properties of arteries from muscles composed mainly of oxidative or mainly of glycolytic fibers. Such variety provides blood flow in the diaphragm adequate to its functional load with various patterns of activity. The mechanisms of vasoregulation in the diaphragm can qualitatively differ in the proximal and distal parts of the vascular bed. The functional properties of the proximal arteries can in part be explained by their proximity to the aorta and their small length. The contractile characteristics and blood supply of the diaphragm in various conditions should be considered when conducting respiratory muscle training in sports and rehabilitation medicine.
This is a preview of subscription content, log in via an institution to check access.
Access this article
Subscribe and save.
- Get 10 units per month
- Download Article/Chapter or eBook
- 1 Unit = 1 Article or 1 Chapter
- Cancel anytime
Price includes VAT (Russian Federation)
Instant access to the full article PDF.
Rent this article via DeepDyve
Institutional subscriptions
Similar content being viewed by others
Work of Breathing During Exercise: Implications for Performance
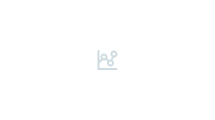
Coronary and muscle blood flow during physical exercise in humans; heterogenic alliance
Strategies of adaptation of small arteries in diaphragm and gastrocnemius muscle to aerobic exercise training.
Fogarty, M.J. and Sieck, G.C., Evolution and functional differentiation of the diaphragm muscle of mammals, Compr. Physiol., 2019, vol. 9, no. 2, pp. 715–766.
PubMed PubMed Central Google Scholar
Manohar, M., Costal vs. crural diaphragmatic blood flow during submaximal and near-maximal exercise in ponies, J. Appl. Physiol., 1988, vol. 65, no. 4, pp. 1514–1519.
CAS PubMed Google Scholar
Kirkton, S.D., Howlett, R.A., Gonzalez, N.C., Giuliano, P.G., Britton, S.L., Koch, L.G., Wagner, H.E., and Wagner, P.D., Continued artificial selection for running endurance in rats is associated with improved lung function, J. Appl. Physiol., 2009, vol. 106, no. 6, pp. 1810–1818.
Rosser-Stanford, B., Backx, K., Lord, R., and Williams, E.M., Static and dynamic lung volumes in swimmers and their ventilatory response to maximal exercise, Lung, 2019, vol. 197, no. 1, pp. 15–19.
PubMed Google Scholar
Neder, J.A., Dal, CorsoS., Malaguti, C., Reis, S., De Fuccio, M.B., Schmidt, H., Fuld, J.P., and Nery, L.E., The pattern and timing of breathing during incremental exercise: A normative study, Eur. Respir. J., 2003, vol. 21, no. 3, pp. 530–538.
Fogarty, M.J., Mantilla, C.B., and Sieck, G.C., Breathing: Motor control of diaphragm muscle, Physiology (Bethesda), 2018, vol. 33, no. 2, pp. 113–126.
CAS PubMed PubMed Central Google Scholar
Schiaffino, S. and Reggiani, C., Fiber types in mammalian skeletal muscles, Physiol. Rev., 2011, vol. 91, no. 4, pp. 1447–1531.
Schiaffino, S., Sandri, M., and Murgia, M., Activity-dependent signaling pathways controlling muscle diversity and plasticity, Physiology (Bethesda), 2007, vol. 22, no. 4, pp. 269–278.
Bloemberg, D. and Quadrilatero, J., Rapid determination of myosin heavy chain expression in rat, mouse, and human skeletal muscle using multicolor immunofluorescence analysis, PLoS One, 2012, vol. 7, no. 4.
Delp, M.D. and Duan, C., Composition and size of type I, IIA, IID/X, and IIB fibers and citrate synthase activity of rat muscle, J. Appl. Physiol., 1996, vol. 80, no. 1, pp. 261–270.
Tarasova, O.S., Kalenchuk, V.U., Borzykh, A.A., Andreev-Andrievsky, A.A, Buravkov, S.V., Sharova, A.P., and Vinogradova, O.L., A comparative analysis of the vasomotor responses and innervation of small arteries in rat locomotor and respiratory muscles, Biophysics, 2008, vol. 53, pp. 621–625.
Google Scholar
Borzykh, A.A., Andreev-Andrievskiy, A.A., Kalenchuk, V.U., Mochalov, S.V., Buravkov, S.V., Kuzmin, I.V., Borovik, A.S., Vinogradova, O.L., and Tarasova, O.S., Strategies of adaptation of small arteries in diaphragm and gastrocnemius muscle to aerobic exercise training, Hum. Physiol., 2017, vol. 43, no. 4, pp. 437–445.
CAS Google Scholar
Metzger, J.M., Scheidt, K.B., and Fitts, R.H., Histochemical and physiological characteristics of the rat diaphragm, J. Appl. Physiol., 1985, vol. 58, no. 4, pp. 1085–1091.
Borzykh, A.A., Gainullina, D.K., Kuz’min, I.V., Sharova, A.P., Tarasova, O.S., and Vinogradova, O.L., Comparative analysis of gene expression in locomotor muscles and rat diaphragm, Ross. Fiziol. Zh. im. I.M. Sechenova, 2012, vol. 98, no. 12, pp. 1587–1594.
Uribe, J.M., Stump, C.S., Tipton, C.M., and Fregosi, R.F., Influence of exercise training on the oxidative capacity of rat abdominal muscles, Respir. Physiol., 1992, vol. 88, nos. 1–2, pp. 171–180.
Metzger, J.M. and Fitts, R.H., Contractile and biochemical properties of diaphragm: Effects of exercise training and fatigue, J. Appl. Physiol., 1986, vol. 60, no. 5, pp. 1752–1758.
Popov, D.V., Adaptation of skeletal muscles to contractile activity of varying duration and intensity: The role of PGC-1α, Biochemistry (Moscow), 2018, vol. 83, no. 6, pp. 613–628.
Suzuki, J., Microvascular remodelling after endurance training with Co 2+ treatment in the rat diaphragm and hind-leg muscles, Jpn. J. Physiol., 2002, vol. 52, no. 5, pp. 409–419.
Gute, D., Fraga, C., Laughlin, M.H., and Amann, J.F., Regional changes in capillary supply in skeletal muscle of high-intensity endurance-trained rats, J. Appl. Physiol., 1996, vol. 81, no. 2, pp. 619–626.
Green, H.J., Plyley, M.J., Smith, D.M., and Kile, J.G., Extreme endurance training and fiber type adaptation in rat diaphragm, J. Appl. Physiol., 1989, vol. 66, no. 4, pp. 1914–1920.
Gosselin, L.E., Betlach, M., Vailas, A.C., and Thomas, D.P., Training-induced alterations in young and senescent rat diaphragm muscle, J. Appl. Physiol., 1992, vol. 72, no. 4, pp. 1506–1511.
Domínguez-Álvarez, M., Gea, J., and Barreiro, E., Inflammatory events and oxidant production in the diaphragm, gastrocnemius, and blood of rats exposed to chronic intermittent hypoxia: therapeutic strategies, J. Cell Physiol., 2017, vol. 232, no. 5, pp. 1165–1175.
Armstrong, R.B. and Laughlin, M.H., Metabolic indicators of fibre recruitment in mammalian muscles during locomotion, J. Exp. Biol., 1985, vol. 115, pp. 201–213.
Joyner, M.J. and Casey, D.P., Regulation of increased blood flow (hyperemia) to muscles during exercise: A hierarchy of competing physiological needs, Physiol. Rev., 2015, vol. 95, no. 2, pp. 549–601.
Murrant, C.L. and Sarelius, I.H., Local control of blood flow during active hyperaemia: What kinds of integration are important?, J. Physiol., 2015, vol. 593, no. 21, pp. 4699–4711.
Sexton, W.L. and Poole, D.C., Costal diaphragm blood flow heterogeneity at rest and during exercise, Respir. Physiol., 1995, vol. 101, no. 2, pp. 171–182.
Laughlin, M.H. and Armstrong, R.B., Rat muscle blood flows as a function of time during prolonged slow treadmill exercise, Am. J. Physiol., 1983, vol. 244, no. 6, pp. H814–824.
Sarelius, I. and Pohl, U., Control of muscle blood flow during exercise: Local factors and integrative mechanisms, Acta Physiol. (Oxford), 2010, vol. 199, no. 4, pp. 349–365.
Bagher, P. and Segal, S.S., Regulation of blood flow in the microcirculation: Role of conducted vasodilation, Acta Physiol., 2011, vol. 202, no. 3, pp. 271–284.
Mel'kumyants, A.M., On the role of endothelial glycocalyx in the mechanogenic regulation of arterial resistance, Usp. Fiziol. Nauk, 2012, vol. 43, no. 4, pp. 45–58.
Fixler, D.E., Atkins, J.M., Mitchell, J.H., and Horwitz, L.D., Blood flow to respiratory, cardiac, and limb muscles in dogs during graded exercise, Am. J. Physiol., 1976, vol. 231, no. 5, pp. 1515–1519.
Manohar, M., Inspiratory and expiratory muscle perfusion in maximally exercised ponies, J. Appl. Physiol., 1990, vol. 68, no. 2, pp. 544–548.
Nobrega, A.C.L., O’Leary, D., Silva, B.M., Marongiu, E., Piepoli, M.F., and Crisafulli, A., Neural regulation of cardiovascular response to exercise: Role of central command and peripheral afferents, Biomed Res. Int., 2014, vol. 2014, p. 478965.
O’Leary, D.S., Robinson, E.D., and Butler, J.L., Is active skeletal muscle functionally vasoconstricted during dynamic exercise in conscious dogs?, Am. J. Physiol., 1997, vol. 272, no. 1, pp. R386–R391.
Manohar, M., Vasodilator reserve in respiratory muscles during maximal exertion in ponies, J. Appl. Physiol., 1986, vol. 60, no. 5, pp. 1571–1577.
Sheel, A.W., Boushel, R., and Dempsey, J.A., Competition for blood flow distribution between respiratory and locomotor muscles: Implications for muscle fatigue, J. Appl. Physiol., 2018, vol. 125, no. 3, pp. 820–831.
Dempsey, J.A., Romer, L., Rodman, J., Miller, J., and Smith, C., Consequences of exercise-induced respiratory muscle work, Respir. Physiol. Neurobiol., 2006, vol. 151, nos. 2–3, pp. 242–250.
Laughlin, M.H. and Armstrong, R.B., Adrenoreceptor effects on rat muscle blood flow during treadmill exercise, J. Appl. Physiol., 1987, vol. 62, no. 4, pp. 1465–1472.
Behnke, B.J., Armstrong, R.B., and Delp, M.D., Adrenergic control of vascular resistance varies in muscles composed of different fiber types: Influence of the vascular endothelium, Am. J. Physiol. Regul. Integr. Comp. Physiol., 2011, vol. 301, no. 3, pp. R783–R790.
Hilton, S.M., Jeffries, M.G., and Vrbová, G., Functional specializations of the vascular bed of soleus, J. Physiol., 1970, vol. 206, no. 3, pp. 543–562.
Aaker, A. and Laughlin, M.H., Diaphragm arterioles are less responsive to alpha1-adrenergic constriction than gastrocnemius arterioles, J. Appl. Physiol., 2002, vol. 92, no. 5, pp. 1808–1816.
Laughlin, M.H., Woodman, C.R., Schrage, W.G., Gute, D., and Price, E.M., Interval sprint training enhances endothelial function and eNOS content in some arteries that perfuse white gastrocnemius muscle, J. Appl. Physiol., 2004, vol. 96, no. 1, pp. 233–244.
Van Teeffelen, J.W.G.E. and Segal, S.S., Interaction between sympathetic nerve activation and muscle fibre contraction in resistance vessels of hamster retractor muscle, J. Physiol., 2003, vol. 550, no. 2, pp. 563–574.
McCurdy, M.R., Colleran, P.N., Muller-Delp, J., and Delp, M.D., Effects of fiber composition and hindlimb unloading on the vasodilator properties of skeletal muscle arterioles, J. Appl. Physiol., 2000, vol. 89, no. 1, pp. 398–405.
Schwartz, L.M. and McKenzie, J.E., Adenosine and active hyperemia in soleus and gracilis muscle of cats, Am. J. Physiol., 1990, vol. 259, no. 4, pp. H1295–H1304.
Muller-Delp, J.M., Spier, S.A., Ramsey, M.W., and Delp, M.D., Aging impairs endothelium-dependent vasodilation in rat skeletal muscle arterioles, Am. J. Physiol. Heart Circ. Physiol., 2002, vol. 283, no. 4, pp. H1662–H1672.
Aaker, A. and Laughlin, M.H., Differential adenosine sensitivity of diaphragm and skeletal muscle arterioles, J. Appl. Physiol., 2002, vol. 93, no. 3, pp. 848–856.
Gainullina, D.K., Kiryukhina, O.O., and Tarasova, O.S., Nitric oxide in vascular endothelium: Production regulation and mechanisms of action, Usp. Fiziol. Nauk, 2013, vol. 44, no. 4, pp. 88–102.
McAllister, R.M., Endothelium-dependent vasodilation in different rat hindlimb skeletal muscles, J. Appl. Physiol., 2003, vol. 94, no. 5, pp. 1777–1784.
Copp, S.W., Holdsworth, C.T., Ferguson, S.K., Hirai, D.M., Poole, D.C., and Musch, T.I., Muscle fibre-type dependence of neuronal nitric oxide synthase-mediated vascular control in the rat during high speed treadmill running, J. Physiol., 2013, vol. 591, no. 11, pp. 2885–2896.
Shipley, R.D., Kim, S.J., and Muller-Delp, J.M., Time course of flow-induced vasodilation in skeletal muscle: Contributions of dilator and constrictor mechanisms, Am. J. Physiol. Heart Circ. Physiol., 2005, vol. 288, no. 4, pp. H1499–H1507.
Aleksandrova, N.P., Baranov, V.M., Tikhonov, M.A., Kolesnikov, V.I., Kotov, A.N., and Kochanov, V.S., Effect of antiorthostatic hypokinesia on the functional state of the diaphragm in rats, Ross. Fiziol. Zh. im. I.M. Sechenova, 2005, vol. 91, no. 11, pp. 1312–1319.
Neder, J.A., Marillier, M., Bernard, A., Matthew, J.D., Kathryn, M.M., and O’Donnell, D.E., The integrative physiology of exercise training in patients with COPD, COPD, 2019, vol. 16, no. 2, pp. 182–195.
Illi, S.K., Held, U., Frank, I., and Spengler, C.M., Effect of respiratory muscle training on exercise performance in healthy individuals: A systematic review and meta-analysis, Sports Med., 2012, vol. 42, no. 8, pp. 707–724.
Download references
The review was written with the financial support of the Russian Science Foundation (project no. 19-75-00060).
Author information
Authors and affiliations.
Institute for Biomedical Problems, Russian Academy of Sciences, 123007, Moscow, Russia
A. A. Borzykh, O. L. Vinogradova & O. S. Tarasova
Faculty of Basic Medicine, Moscow State University, 119991, Moscow, Russia
O. L. Vinogradova
Faculty of Biology, Moscow State University, 119234, Moscow, Russia
O. S. Tarasova
You can also search for this author in PubMed Google Scholar
Corresponding author
Correspondence to A. A. Borzykh .
Ethics declarations
The authors declare that they have no conflict of interest. This article does not contain any studies involving animals or human participants performed by any of the authors.
Additional information
Translated by I. Shipounova
About this article
Borzykh, A.A., Vinogradova, O.L. & Tarasova, O.S. Diaphragm: The Relationship between Blood Supply Regulation and Characteristics of the Contractile Function. Moscow Univ. Biol.Sci. Bull. 75 , 41–49 (2020). https://doi.org/10.3103/S0096392520020029
Download citation
Received : 22 March 2020
Revised : 05 May 2020
Accepted : 17 May 2020
Published : 15 September 2020
Issue Date : April 2020
DOI : https://doi.org/10.3103/S0096392520020029
Share this article
Anyone you share the following link with will be able to read this content:
Sorry, a shareable link is not currently available for this article.
Provided by the Springer Nature SharedIt content-sharing initiative
- myosin heavy chain isoforms
- metabolic types of muscle fibers
- respiratory muscles
- resistance arteries
- exercise hyperemia
- sympathetic nervous system
- nitric oxide
- Find a journal
- Publish with us
- Track your research
Metastasis of endometrial cancer to right hemidiaphragm: A case report
Affiliations.
- 1 A.V. Vishnevsky National Medical Research Centre of Surgery, Bolshaya Serpukhovskaya str. 27, 117997, Moscow, Russia. Electronic address: [email protected].
- 2 Research and Practical Clinical Centre of Diagnostics and Telemedicine Technologies, st. Srednyaya Kalitnikovskaya str. 28, 109029, Moscow, Russia. Electronic address: [email protected].
- 3 A.V. Vishnevsky National Medical Research Centre of Surgery, Bolshaya Serpukhovskaya str. 27, 117997, Moscow, Russia. Electronic address: [email protected].
- 4 A.V. Vishnevsky National Medical Research Centre of Surgery, Bolshaya Serpukhovskaya str. 27, 117997, Moscow, Russia.
- 5 Department of Thoracic Surgery, A.V. Vishnevsky National Medical Research Centre of surgery, Bolshaya Serpukhovskaya str. 27, 117997, Moscow, Russia.
- 6 V. Vishnevsky National Medical Research Centre of surgery, Pirogov Russian National Research Medical University, Bolshaya Serpukhovskaya str. 27, 117997, Moscow, Russia. Electronic address: [email protected].
- PMID: 31288200
- PMCID: PMC6614545
- DOI: 10.1016/j.ijscr.2019.06.028
Introduction: Tumors of the diaphragm are uncommon. The overwhelming number of cases is metastatic combined with metastases to the liver, lungs and other organs. Only a minority of cases are described as solitary lesions.
Case presentation: Fifty-five years old female with a history of radical curative surgery for pT3N0M0 endometrial cancer eight years ago was admitted to the Department of Thoracic Surgery with a feeling of discomfort in the right hypochondrium. The contrast-enhanced MDCT revealed a large, well-circumscribed lesion of the right hemidiaphragm deforming upper contour of the liver. A clear boundary between the lesion and the liver suggested former's diaphragmatic origin. PET-CT did not show any distant metastasis. Intraoperative revision revealed a tumor growing from the dome of the diaphragm with well-defined contours and without any signs of lung involvement. The diaphragmotomy was performed. The morphological study with immunohistochemistry showed an endometrial carcinoma metastasis to the diaphragm.
Conclusion: The diaphragm lesions can have various etiology, but a probability of tumor metastasis after a previous radical surgery should not be excluded. Preoperative differential diagnostics can be difficult, leaving surgical treatment followed by a pathology study as the most informative diagnostic method of tumor morphology.
Keywords: CT; Diaphragmotomy; Endometrial cancer; Metastasis to the diaphragm.
Copyright © 2019 The Authors. Published by Elsevier Ltd.. All rights reserved.
Maps: Ukraine's incursion into Russia forces Moscow to make an important decision
Ukraine's shock incursion across the Russian border into Kursk Oblast may force important strategic decisions on Moscow as President Vladimir Putin's troops are taken as prisoners of war and supply lines are threatened. The Ukrainian attack took Russian forces by surprise, according to one U.S. official who was not authorized to speak publicly .
According to the Institute for the Study of War , Ukraine’s cross-border gambit allowed Kyiv to seize the battlefield initiative, long held by Russian forces who were able to dictate the time and place of fighting and force Ukrainian troops to expend manpower and equipment on defensive operations.
“It's been a very real success,” former U.S. Ambassador to Ukraine John Herbst, now a senior director at the Atlantic Council , told USA TODAY. “The latest data, not confirmed, says they've taken as much as 750 square kilometers (289.5 square miles) and may have gone as far as 35 kilometers (21.75 miles) from the border.”
One week in Kursk : See evolution of Ukraine's incursion across Russian border
Can't see the map above ? Click here to reload the page.
The war institute says Putin may have incorrectly assessed that Ukraine did not have the capacity for such an attack − and that Russia’s treatment of the international border as a dormant front line represents a strategic failure.
Seth Jones, director of the International Security Program at the Center for Strategic and International Studies told USA TODAY that the attack was a "huge psychological success and morale success for the Ukrainians because it puts the Russians on their back foot. It surprised the Russian military. It makes them look incompetent for not protecting their border, and I think it's a huge morale boost to a Ukraine that's really needed something recently, even though it's had successes in the Black Sea, including against the Russian Navy and in Crimea."
"The tables have been turned, at least slightly and at least temporarily, with the Ukrainian incursion," said Jones, "This is a Ukrainian offensive operation. It looks very different from those we saw in 2023 or 2022, which were trying to retake territory that the Russians had seized in Ukraine. This is moving into Russian territory, which I think took the Russians by surprise. This was a real surprise attack."
The push into Kursk may force Russia into a decision to treat the border as a front rather than a dormant area, with implications for Moscow's deployment of personnel and materiel within Ukraine. The war study institute reports that Russian defensive forces appear to be hastily assembled and may be below intended strength and that Russian conscripts stationed in Kursk were unable to retreat and return to their units. The capture or death of conscripts poses a political risk to Putin because he would need to explain the casualties to the Russian public, according to the war study institute.
"It's almost certain that the Russians are reconsidering force deployments, but reconsidering is not the same as deciding to change those deployments in a major way," Herbst said.
"Russia, politically, can't afford to let this happen again," said Jones, "I think it'll force them both to keep an eye on intelligence indications and warnings and also devote more forces to protecting the border."
A video posted online showed a "heavily damaged" Russian convoy in Kursk filmed early Friday, according to BBC Verify . The uploader was later arrested by Russian authorities.
Herbst said that “Russian efforts to stop (Ukrainian forces) have so far been at best fruitless and at worst disastrous. The destruction of that armor column a few days ago was a disaster. There are still not enough Russian forces to stop this offensive.” But Herbst cautioned that more Russian personnel and equipment may be headed to the area and may be able to resist Ukrainian forces.
Reuters reports that Russian tanks, artillery and rocket systems were moved to the southern Kursk region as Moscow "imposed anti-terrorism measures" .
The operation could present a real problem for Putin according to Herbst, who suggests the Russian leader may be downplaying the severity of the incursion by declaring a counterterrorism operation . "A counterterrorism operation is not the same as a war where you have opposing troops on your soil, which of course is what you have here."
The incursion comes as prominent Russian oligarchs are speaking out against the war in Ukraine. In an interview with Nikkei Asia , Oleg Deripaska, a billionaire metal magnate with close ties to Putin, called the war "mad" and criticized Russia's defense spending. "If you want to stop the war, first you need to stop the fire," he said, calling for an "immediate, unconditional cease-fire" in Ukraine.
Deripaska "does not seem to be a candidate for defenestration, so watching what happens to him and watching to see if there will be other voices like that could be an indication of things coming apart in Moscow," said Herbst, referring to a long string of suspicious deaths of Kremlin critics.
According to The Institute for the Study of War , the Kremlin released a half-hour video of Putin reprimanding federal and regional officials with the apparent intention of sending a message to other government officials to refrain from commenting on Ukraine's Kursk incursion.
Ukrainian President Volodymyr Zelenskyy acknowledged the operation in a post Saturday to X, formerly Twitter , describing a push to drive the war into "the aggressor's territory." Zelenskyy thanked international partners for implementing sanctions against Russia and the United States for new defense aid, including Stinger missiles, HIMARS mobile artillery ammunition and 155mm artillery shells.
Beyond Kursk, Jones said Russia's main effort for offensive operations has been Eastern Ukraine. "It's been primarily to increase control of areas. We've seen a big push in Luhansk Oblast," said Jones, "The challenge has been they haven't been able to take areas quickly and they haven't been able to take advantage of changes in strategy. So they're taking huge casualties when they do it."
"Huge casualties to the degree that were somewhere around 500,000 total casualties since the war began, which is enormous. These are astronomical numbers that Russia or the Soviet Union haven't faced since World War II."
Read more about the war in Ukraine:
- One week in Kursk: Maps show Ukraine offensive as Russia builds trenches after attack
- What's behind Russia's sluggish response to Ukrainian raid?
- Ukrainian attack edges into Russian city, site of pipeline, railroad
- After Ukraine troops cross into Kursk, Putin blasts 'provocation'
- From 2023: Maps of Ukraine counteroffensive and Russian defenses show advances, battle lines
- From 2022: Fighting rages around Europe's biggest nuclear power plant
Keep up with news abroad and its impact in the US: Sign up for USA TODAY's Russia-Ukraine War newsletter.
Contributing: Carlie Procell, Tom Vanden Brook and Dan Morrison

An official website of the United States government
The .gov means it’s official. Federal government websites often end in .gov or .mil. Before sharing sensitive information, make sure you’re on a federal government site.
The site is secure. The https:// ensures that you are connecting to the official website and that any information you provide is encrypted and transmitted securely.
- Publications
- Account settings
Preview improvements coming to the PMC website in October 2024. Learn More or Try it out now .
- Advanced Search
- Journal List
- J Thorac Dis
- v.16(5); 2024 May 31
- PMC11170431
Thoracic manifestations and respiratory function alterations in axial spondyloarthritis and newest possibilities of ultrasound to detect changes in diaphragm—a narrative review
Hana skala kavanagh.
1 University Department of Rheumatology, Physical and Rehabilitation Medicine, University Clinical Centre Sestre Milosrdnice, Zagreb, Croatia;
Jan Aksentijević
2 Department of Physical and Rehabilitation Medicine with Rheumatology, University Hospital Dubrava, Zagreb, Croatia;
Frane Grubišić
Simeon grazio, marija gomerčić palčić.
3 Division of Pulmonology, University Department for Internal Diseases, University Clinical Centre Sestre Milosrdnice, Zagreb, Croatia
Associated Data
The article’s supplementary files as
Background and Objective
Axial spondyloarthritis (axSpA) includes thoracic manifestations and changes in respiratory function that require a comprehensive understanding for effective treatment. This review aims to investigate these manifestations and evaluate the role of ultrasound in detecting diaphragmatic changes to provide insights for improved diagnosis and treatment strategies in axSpA patients.
A systematic search was conducted in Index Medicus and Scopus from 2003 to 2023. Inclusion criteria included primary and secondary publications, with a focus on high-quality evidence such as randomised controlled trials and systematic reviews with or without meta-analysis. Keywords spondyloarthritis, respiratory, chest, thoracic, diaphragm and ultrasound were used in the search. A total of 22 articles were identified after duplicates, and inadequate papers were removed.
Key Content and Findings
The review included the prevalence, classification and extra-articular manifestations of axSpA, highlighting the impact on respiratory function. Thoracic manifestations and the potential impact of pharmacological interventions were detailed, and various conditions affecting respiratory dynamics were discussed. In addition, the utility of ultrasonography in assessing diaphragmatic function was explained and the techniques, parameters and measurements used to assess diaphragmatic movement, muscle thickness and respiratory mobility were described. The results illustrate the changes in diaphragmatic function in axSpA patients and their correlation with disease activity.
Conclusions
This narrative review highlights the intricate relationship between axSpA and respiratory manifestations and emphasises the significant impact on thoracic function and diaphragmatic dynamics. The utility of ultrasound in assessing diaphragmatic function offers a promising avenue for objective evaluation that provides insight into disease activity and potential therapeutic responses. This review emphasises the critical role of early diagnosis and vigilant monitoring, and advocates a multidisciplinary approach that integrates non-pharmacological interventions, particularly tailored physical activity, to maintain and improve respiratory function in axSpA patients. Increased research initiatives and awareness of pulmonary complications in axSpA are essential to optimise medical care and improve treatment outcomes in this patient group.
Introduction
Axial spondyloarthritis (axSpA) is a chronic inflammatory rheumatic disease predominantly affecting the spine and sacroiliac joints. The clinical picture is characterised by pain, stiffness and limited mobility with a resulting restriction of functional capacity. In 2009, the Assessment of SpondyloArthritis International Society (ASAS) proposed classification criteria for axSpA ( 1 ). Taking into account the presence of changes on native radiograph or magnetic resonance only, the nomenclature distinguishes between radiographic axSpA (r-axSpA), including ankylosing spondylitis (AS), and non-radiographic axSpA (nr-axSpA) ( 2 ). The estimated prevalence of axSpA is 0.36% to 0.70% and AS between 0.20% and 0.25% ( 3 , 4 ).
In axSpA, there are often extra-articular manifestations of the disease that are part of the SpA concept, but also those that are not. Considering the organ system affected, they can be divided into: ocular manifestations (uveitis—mostly unilateral, acute, anterior uveitis) ( 5 ), gastrointestinal (inflammatory bowel disease) ( 6 , 7 ), skin (psoriasis) ( 8 ), bone (osteoporosis and associated low energy vertebral fractures) ( 9 ), cardiovascular (conduction disorders, valvular heart disease, cardiomyopathy, atherosclerosis, thrombosis, aortitis, pericarditis, vasculitis) ( 10 - 13 ), renal disease (IgA glomerulonephritis, amyloid deposition, microhaematuria, microalbuminuria, impaired renal function) ( 14 ) and pulmonary manifestations, described in detail later.
Disease assessment in people with axSpA is a comprehensive and multi-layered process that includes dimensions such as disease activity, functional status, pain perception, stiffness, fatigue, mobility and quality of life. The ASAS/OMERACT (Outcome Measures in Rheumatology) consortium has provided recommendations for assessing outcomes in this context ( 15 ).
Treatment modalities include both pharmacological and non-pharmacological interventions. Despite the remarkable progress made by the widespread use of biologics, which have significantly improved outcomes in this group, a subgroup of patients show suboptimal response or intolerance to these therapies ( 16 ). For all patients, especially this subgroup, it is of utmost importance to achieve positive treatment outcomes through lifestyle modifications. The European Alliance of Associations for Rheumatology (EULAR), along with most national rheumatology societies, advocates the inclusion of regular physical activity as it is associated with reduced disease activity, slowing of disease progression, attenuation of functional impairment, improved thoracic and spinal mobility, improved cardiorespiratory function and overall improvement in well-being ( 17 , 18 ).
Assessment of physical function is recognised as a critical aspect within the ASAS/OMERACT core collection of outcome measures. However, despite the need for a holistic approach to the treatment of axSpA, involving a harmonious integration of pharmacological and non-pharmacological approaches, the latter interventions are often undervalued and neglected. This dilemma presents a particular challenge, as empirical clinical research undeniably highlights the limited capacity for physical activity in individuals with axSpA ( 19 - 21 ). We present this article in accordance with the Narrative Review reporting checklist (available at https://jtd.amegroups.com/article/view/10.21037/jtd-23-1936/rc ).
The aim of this paper is to review the thoracic manifestations and changes in respiratory function in axSpA, and to gain insight into the latest ultrasound capabilities to detect changes in the diaphragm in these patients that may potentially impact early diagnosis and treatment outcomes.
To this end, we conducted a review of the existing literature in two major databases, Index Medicus and Scopus, from 2003 to 2023. Our search included secondary and tertiary publications, focusing on the highest ranked articles according to the evidence-based medicine hierarchy, randomised controlled trials and systematic literature reviews with or without meta-analysis. Using the keywords spondyloarthritis, respiratory, chest, thoracic, diaphragm and ultrasound, we initially found 242 articles. After subtracting duplicates and papers that do not match thematically, there were 22 that are included in this narrative review. An overview of the database research strategy is presented in Table 1 .
Thoracic manifestations and respiratory function alterations in axSpA
The first descriptions of lung and pleural involvement in individuals with spondyloarthritis date back to 1941 and 1949 ( 22 ). Although changes in lung function and ventilatory disturbances are often due to changes in the stiffness and elasticity of the thorax and thoracic spine, it is important to highlight the conditions stemming from pulmonary parenchymal changes, as well as those that occur as potential consequences of pharmacological interventions in axSpA. The predominant clinical conditions that lead to impaired respiratory function in axSpA patients include chest discomfort, restrictive chest disorders, interstitial lung disease, apical fibrosis (apical fibrobulous disease), pulmonary superinfection, spontaneous pneumothorax, obstructive sleep apnoea, bronchiolitis obliterans, bronchiocentric granulomatosis, cricoarytenoid arthritis and iatrogenic reactivation of latent tuberculosis ( 23 , 24 ).
In addition to reactivation of latent tuberculosis [often associated with anti-tumor necrosis factor (TNF)-α drugs], pneumonitis (associated with methotrexate, leflunomide and gold salts), DRESS (drug reaction with eosinophilia and systemic symptoms) associated with sulfasalazine, asthma, triggered by nonsteroidal anti-inflammatory drugs (NSAIDs), and rare cases of new-onset, and progression of interstitial lung disease (reported with anti-TNF-α drugs) are possible side effects of pharmacological interventions for inflammatory rheumatological diseases ( 25 ). Pleuritis and lupus-like syndrome (associated with anti-TNF-α drugs) further expand the spectrum of lung involvement ( 25 ). In addition, the prevalence of chronic obstructive pulmonary disease (COPD) is significantly higher in people with psoriatic arthritis and AS ( 26 ).
Inflammatory changes in the joints and entheses, coupled with ossification and fusion of the thoracic spine, costovertebral joints and occasionally costosternal joints, sternoclavicular joints and manubriosternal synchondrosis contribute to a reduction in chest mobility. These changes culminate in the development of thoracic kyphosis, inflexibility of the thorax, formation of syndesmophytes and further decrease in spinal and thoracic mobility during the respiratory cycle ( 27 ). The persistent stiffness of the rib cage exacerbates atrophy of the intercostal muscles, which increases the workload on the diaphragm as the main inspiratory muscle. All these factors contribute to a reduction in lung volume and capacity compared to the healthy population ( 28 - 30 ).
Fibrocystic changes in the upper lobe of the lung are thought to result from thickening of the apical portion of the pleura due to decreased ventilation of the upper lobes of the lung as a result of chest stiffness ( 31 ). Although these changes are predominantly asymptomatic, they can lead to superinfections with mycobacteria or fungi, especially Aspergillus fumigatus . Furthermore, they can occasionally lead to unilateral or, rarely, bilateral spontaneous pneumothorax (occurring in about 0.29% of patients), which requires immediate medical attention ( 32 ).
The gold standard for diagnosing restrictive lung disease is measurement of total lung capacity (TLC) by plethysmography. However, due to its limited availability in certain healthcare settings, spirometry is recommended as a reliable screening tool to identify a restrictive pattern in respiratory dysfunction with a sensitivity of about 60% ( 29 , 33 , 34 ).
In individuals affected by AS, the decline in lung function typically manifests as a restrictive pattern, with a prevalence of 20–57% ( 35 ). This phenomenon is primarily due to restricted chest mobility, which is present in almost all individuals with advanced disease, and to a lesser extent, interstitial lung disease ( 24 , 36 - 40 ).
Although restrictive pulmonary changes in axSpA are often asymptomatic, their impact on functionality and quality of life is undeniable ( 41 ). Studies have revealed a negative correlation between chest mobility and forced vital capacity (FVC) and forced expiratory volume in the first second (FEV1) values ( 28 , 41 - 43 ). A characteristic phenomenon is the maintenance or even enhancement of gas exchange across the alveolar-capillary membrane, which suggests extrapulmonary limitation rather than primary pulmonary dysfunction ( 25 ).
Comparative study by Berdal et al. found a relationship between spinal and thoracic mobility and changes in the percentage of expected FVC in individuals with AS. Of note, the authors found no significant correlations between lung function and measures of disease activity, physical function, smoking and cardiorespiratory functional capacity during physical activity ( 40 ). A study by Romagnoli et al. demonstrated an increased workload of the diaphragm and abdominal muscles in AS patients that compensates for limited chest mobility ( 44 ). In individuals with AS and kyphotic deformities of the thoracic spine, changes in the direction of movement of the diaphragm and a shortened anterior abdominal wall adversely affect the respiratory process. Liu et al. also confirmed that AS patients with kyphotic deformities experience sagittal axial rotation of the diaphragm, which can reduce both vital capacity and FVC ( 45 ).
This underlines the importance of preserving spinal mobility in patients while emphasising that the prevention of radiological progression, starting with the immediate initiation of adequate treatment, must be a top priority.
Ultrasound assessment of diaphragm function and its significance in people with axSpA
The diaphragm, a vital respiratory muscle, has a dome-shaped form and can be anatomically divided into two main segments: the central portion, which consists of a tendon, and the outer muscular portion. Functionally, this muscular part is further divided into two components: the crural segment, located medially and originating from the lumbar vertebrae (L2–L4), and the larger costal segment, located laterally and connected to the inner surfaces of the six lower ribs, thus forming the zone of apposition (ZOA) ( 46 , 47 ).
During breathing, contraction of the muscle fibres in the ZOA causes the diaphragm to move craniocaudally, resulting in an increase in intrathoracic volume and a subsequent decrease in intrathoracic pressure. This mechanism facilitates the inflow of air into the lungs. At the same time, the muscle fibres in the costal portion of the diaphragm elevate the lower chest, contributing to its expansion. In the exhalation phase, the diaphragm relaxes and its upward displacement results from the elastic recoil of the lungs. For sustained, rhythmic and uninterrupted breathing, it is essential that the muscle fibres of the diaphragm have some endurance against fatigue. In the adult human diaphragm, the composition of muscle fibres is 55% type I fibres (which are slow fatigue resistant), 21% type IIA fibres (fast oxidative fibres with intermediate fatigue resistance) and 24% type IIB fibres (fast glycolytic fibres with low fatigue resistance) ( 48 ). Ultrasound is used as a means to assess diaphragmatic function by measuring muscle thickening and respiratory mobility. Two acoustic windows are used in this assessment: the subcostal area (SCA) and the ZOA.
During a diaphragmatic contraction, ultrasound examinations through the SCA window provide insights into the cranio-caudal excursion of the diaphragm. To acquire these images, a low frequency phased array or curved array probe (2–5 MHz) is strategically placed just below the costal arch along the midclavicular and anterior axillary line (for anterior subcostal view) or along the mid and posterior axillary line (for posterior subcostal view). The ultrasound probe is tilted cranially and aligned perpendicular to the diaphragmatic dome. The diaphragm is visualised as a hyperechoic line extending over the liver and spleen, which serve as acoustic windows. During the inspiration phase, the diaphragm should move in the direction of the probe. The examination is performed during different breathing patterns, including quiet breathing, deep breathing and the sniffing manoeuvre ( 49 - 52 ).
At the same time, ZOA window is used to observe muscle thickness and thickening fraction (TF). In the intercostal approach, a 10–15 MHz linear array transducer is placed in cranio-caudal alignment and perpendicular to the skin, typically between the mid-axillary line or the antero-axillary line within the 8th to 11th intercostal spaces. The diaphragm appears as a three-layered structure lying between the pleural and peritoneal membranes. Measurements of the diaphragm are taken in either B or M mode using callipers, with recordings at the end of expiration (expressing functional residual capacity—FRC) and at the end of inspiration (expressing TLC) during deep breathing ( 49 , 53 , 54 ).
Boussuges et al. have demonstrated, in their reproducibility and a repeability study, a high degree of reproducibility by the same examiner (96–94%) and between examiners (95–91%) when measuring the movement of both hemidiaphragms during quiet breathing ( 50 ). In addition, measurement of diaphragm thickness fration in M-mode and B-mode has been shown to be reproducible in a group of 66 healthy subjects with a repeatability coefficient of 0.10–0.15 for M-mode and 0.16–0.26 for B-mode. A repeatability coefficient ≤0.3 was considered acceptable. For less experienced users, ultrasound examination with M-mode is more accurate ( 55 , 56 ).
The respiratory mobility of the diaphragm is determined by the maximum distance the diaphragm travels between forced inspiration and expiration (in millimetres). Normal values of diaphragmatic mobility depend on the patient’s sex, position, positioning and orientation of the ultrasound probe, the side of the hemidiaphragm and the type of inspiration (quiet breathing or deep breathing).
During deep breathing in the supine position, the measurements are as follows:
- ❖ Right hemidiaphragm: 4.7±1.0 cm (female) vs. 5.3±1.1 cm (male);
- ❖ Left hemidiaphragm: 4.8±0.3 cm (female) vs. 5.4±1.3 cm (male).
For comparison, in standing position, the values are:
- ❖ Right hemidiaphragm: 5.7±1.0 cm (female); 7.0±1.1 cm (male);
- ❖ Left hemidiaphragm: 6.4±1.0 cm (female); 7.5±0.9 cm (male).
During quiet breathing, the sex- and position-related differences are significantly smaller, with the following values:
- ❖ Right hemidiaphragm: 1.6±0.3 cm (female); 1.8±0.3 cm (male);
- ❖ Left hemidiaphragm: 1.6±0.4 cm (female); 1.8±0.4 cm (male) ( 50 , 56 ).
Diagnosis of diaphragmatic weakness is established by observing decreased mobility during deep breathing, with or without paradoxical movement during the sniffing manoeuvre ( 57 ).
Assessment of the trophicity and contractile efficiency of the diaphragm involves evaluation of the thickness of the diaphragm (Tdi), the ratio of thickening at the end of inspiration and expiration (TdTLC/TdFRC), and the fraction of diaphragmatic thickening (TF) at the end of forced inspiration (Tdi-insp) and forced expiration (Tdi-exp) [(Dtf) = (tdiTLC − tdiFRC)/tdiFRC] in both hemidiaphragms ( 54 , 58 ).
Tdi-exp provides information about the trophicity of the diaphragm. Reference values for healthy individuals, based on a cross-sectional study of 109 healthy subjects with an average age of 25.8±6.5 years, are 0.14±0.03 cm for women and 0.19±0.04 cm for men ( 59 ).
The lower limit of acceptable diaphragmatic thickness at FRC is 0.15 cm, and an increase in diaphragmatic thickness from FRC to TLC of at least 20% is considered physiological. A difference in thickness from sides at FRC of >0.33 cm is abnormal ( 60 ).
The TF is calculated using the following formula: TF = [(Tdi-insp − Tdi-exp)/Tdi-exp] × 100. Cardenas et al. conducted a study on a sample of 64 healthy participants and determined an average physiological TF of (169±43)% in women and (204±61)% in men ( 52 ).
The diaphragm is expected to thicken by at least 20% during maximal inspiration, with minimal side-to-side variation ( 61 ). It has been found that the displacement of the diaphragm is greater in supination than in standing or sitting, considering the same volume of inspiration ( 62 ). According to Boussuges et al. study on the 410 healthy volunteers the mean value and lower limit of normal diaphragmatic displacement during quiet breathing and the sniffing manoeuvre are comparable to the values for people in a standing position. However, the mean and lower limits of diaphragmatic displacement during deep breathing in the seated position were lower than in the standing position ( 50 ). A technical report by Houston et al. found that the normal right-to-left ratio of maximum diaphragmatic displacements during deep breathing was in the range of 0.5 to 1.6 ( 63 ).
Diagnosis of diaphragmatic dysfunction includes ultrasound values of TF <20–29% and diaphragmatic excursion of less than 1 cm. Reduced, absent or paradoxical diaphragmatic motility supports the diagnosis of diaphragmatic palsy. Reduced Tdi-exp (<0.11 cm) and reduced TF (<20%) support a diagnosis of chronic diaphragmatic palsy, while reduced, absent or paradoxical mobility and reduced TF <20% support a diagnosis of acute diaphragmatic palsy ( 56 ).
Studies have not demonstrated a relationship between diaphragmatic thickness and body mass index (BMI), respiratory index or chest circumference ( 59 ). In contrast to these findings, research by Kantarci et al. on 164 healthy subjects indicates that BMI <18.5 kg/m 2 and >40 kg/m 2 is associated with statistically lower diaphragmatic motility in healthy subjects ( 64 ). Diaphragmatic thickness and contractility are only slightly influenced by age, sex or smoking status ( 60 ).
In people with axSpA, changes in the diaphragm may be seen on ultrasound, indicating muscle dysfunction that could be associated with respiratory symptoms. Ünlü et al. conducted a comparative study with 33 subjects with axSpA and observed a non-significant reduction in diaphragmatic mobility compared to a control group using ultrasound. In addition, their study found a positive correlation between diaphragmatic mobility and the occiput to wall distance and a negative correlation with cervical spine rotation and the modified Schober test. These results suggest a correlation between limited axial mobility and diaphragmatic movement ( 65 ). A cross-sectional study by Dhahri et al. studied diaphragm and respiratory function with ultrasound in 50 people with axSpA. Their study showed a decrease in diaphragm thickness and a correlation between diaphragm thickness and lung function parameters. This was confirmed by the results of spirometry from the same study, which indicated restrictive disorders in 32% of the subjects and pathological values of chest motility in 72%. Diaphragmatic thickness was found to correlate with FVC and supine FVC, further confirming the correlation between ultrasound diaphragmatic assessment and spirometry results ( 66 ).
In a cross-sectional study of 50 patients diagnosed with AS by Mejri Ep Ajili et al. , 54% of the subjects were found to have decreased right hemidiaphragm motility and 48% were found to have decreased left hemidiaphragm motility. In addition, decreased diaphragmatic thickening was found in the right hemidiaphragm in 56% of the subjects and in the left hemidiaphragm in 60%. The study also showed a correlation between right hemidiaphragm inspiratory thickness and FVC and FVC in the supine position ( 67 ).
In a cross-sectional study by Güneş et al. of 49 patients diagnosed with axSpA, a negative correlation was found between the erythrocyte sedimentation score and the diaphragmatic thickness ratio during the respiratory cycle (dtr), which assesses the thickness ratio at maximum inspiration (TLC) and expiration (FRC). This suggests a possible relationship between disease activity and changes in diaphragmatic function. The authors suggested that assessment of dtr could complement pulmonary function tests (PFTs) and emphasised the importance of early interventions, such as specific exercises to improve diaphragmatic function, in people with axial SpA ( 68 ).
Inspiratory muscle training leads to an improvement in inspiratory muscle strength, targeting the diaphragm muscles in particular, thereby increasing functional capacity. This programme also serves to increase chest expansion, which increases its benefit by exerting a positive influence on mitigating disease activity in patients with axSpA ( 69 ).
A thorough understanding of the pulmonary manifestations in people with axSpA is essential for accurate diagnosis and targeted treatment strategies.
A comprehensive, multidisciplinary therapeutic approach that includes careful monitoring of pulmonary changes plays a fundamental role in improving patient outcomes and quality of life.
The use of ultrasound measurements to quantify diaphragm thickness, which, given its central role as the main inspiratory muscle, combined with an assessment of its respiratory mobility, provides objective insights into its functional dynamics. Insufficient diaphragmatic mobility can lead to a reduction in lung volume and capacity, which in turn affects the patient. By detecting inadequate diaphragmatic mobility, ultrasound can alert the physician to a possible reduction in lung volume and capacity so that appropriate measures can be taken to optimise respiratory function. In contrast, the observation of increased diaphragmatic mobility during follow-up examinations can indicate improved disease control and a better response to therapy, so that treatment strategies can be targeted towards a better outcome for the patient. A nuanced understanding of these adaptive changes is essential for ongoing monitoring of the condition of axSpA patients to facilitate therapeutic interventions to optimise respiratory function.
Promoting further research initiatives and raising awareness of pulmonary complications has the potential to improve the efficiency of medical care and treatment outcomes for patients with axSpA.
Supplementary
Acknowledgments.
Funding: None.
Ethical Statement: The authors are accountable for all aspects of the work in ensuring that questions related to the accuracy or integrity of any part of the work are appropriately investigated and resolved.
Reporting Checklist: The authors have completed the Narrative Review reporting checklist. Available at https://jtd.amegroups.com/article/view/10.21037/jtd-23-1936/rc
Peer Review File: Available at https://jtd.amegroups.com/article/view/10.21037/jtd-23-1936/prf
Conflicts of Interest: All authors have completed the ICMJE uniform disclosure form (available at https://jtd.amegroups.com/article/view/10.21037/jtd-23-1936/coif ). The authors have no conflicts of interest to declare.
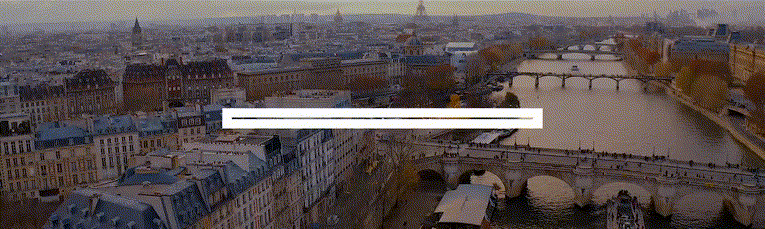
IMAGES
COMMENTS
Decreased diaphragmatic excursion may be detected by percussion of the lower rib cage at end expiration and end inspiration. ... Guillot, F. Surgical treatment of diaphragmatic eventration using ...
The diaphragm is the main inspiratory muscle, and its dysfunction can lead to significant adverse clinical consequences. The aim of this review is to provide clinicians with an overview of the main causes of uni- and bi-lateral diaphragm dysfunction, explore the clinical and physiological consequences of the disease on lung function, exercise physiology and sleep and review the available ...
Furthermore, diaphragmatic excursion is an index for respiratory muscle fatigue during the SBT. Some authors had reported a lower accuracy for diaphragmatic excursion compared to most of the available data and suggested that this lower accuracy is due to the heterogeneity of the patients included in the meta-analyses , . Therefore, separate ...
The diaphragm is a vital organ for mammals as it is the primary muscle for respiration. Diaphragmatic paralysis is the loss of its muscular power and can arise from either weakness of the muscle itself or damage to its nerve supply. Depending on the severity of the paralysis and whether it is unilateral or bilateral, patients can have varied clinical manifestations. A patient may be ...
Although etiology and pathology of symptomatic diaphragm paralysis and eventration are distinct, their treatments are the same: to reduce dysfunctional caudal excursion of the diaphragm during inspiration by plication. Minimally invasive diaphragm plication techniques have emerged as equally effective and less morbid alternatives to open plication. This review focuses on the etiology ...
In patients with chronic obstructive pulmonary disease (COPD), the maximum level of diaphragm excursion (DEmax) is correlated with dynamic lung hyperinflation and exercise tolerance. This study aimed to elucidate the utility of DEmax to predict the improvement in exercise tolerance after pulmonary rehabilitation (PR) in patients with COPD. This was a prospective cohort study.
Diaphragmatic excursion. Diaphragmatic excursion is the movement of the thoracic diaphragm during breathing. Normal diaphragmatic excursion should be 3-5 cm, but can be increased in well-conditioned persons to 7-8 cm. This measures the contraction of the diaphragm. It is performed by asking the patient to exhale and hold it.
Normal diaphragm excursion is between 3 and 5 cm. An abnormal sniff test is the paradoxic mo-tion of a hemidiaphragm upward by greater than ... Surgical plication is the treatment of choice for both symptomatic eventration and diaphragm paralysis. First described in the early twentieth century by Wood and Morrison18, ...
Diaphragmatic dysfunction. The term diaphragmatic dysfunction includes eventration, weakness and diaphragmatic paralysis. 5 Eventration is a permanent elevation of all or part of the hemidiaphragm caused by thinning. 5,6 Diaphragmatic weakness would be the partial loss of muscle strength to generate the necessary pressure for adequate ventilation, 6,7 while paralysis means the total absence of ...
Diaphragmatic weakness or paralysis is caused by damage or pressure on the phrenic nerve. There are several known causes that can lead to diaphragm paralysis: Cancer that has spread and compresses the phrenic nerve. While the cause can be identified in some cases, as many as 40 to 50% of paralyzed diaphragms are idiopathic, meaning the cause is ...
Diaphragmatic function and BMI (body mass index) To evaluate the function of the diaphragm muscle [], the diaphragmatic excursion was measured at rest and during forced expiration (Supplement Table 1).In 60 patients, diaphragmatic excursion at rest in the supine position was 3.5 cm ± 1.2 on the right side and 3.5 cm ± 1.2 on the left side.
Figure 7. Exhalation time of diaphragmatic excursion 3.1 Specific results of diaphragmatic excursion by ultrasound. The mean diaphragmatic thickness measured during expiration was 3.4 mm, while in inspiration it was 2.58 mm; with a thickening fraction of approximately 43 %. The mean diaphragmatic excursion
Introduction. The diaphragm is the main muscle of respiration [].Diaphragmatic excursion is 1-2 cm during tidal breathing and 7-11 cm during deep inspiration [].The assessment of diaphragmatic function is important for diagnosis and follow up of various physiologic and pathologic conditions [].Several methods exist for the evaluation of diaphragmatic function.
Results: In the diaphragmatic stretch technique, there was a statistically significant improvement in the diaphragmatic excursion before and after the treatment. On the right side, p=0.00 and p=0.003 in the midclavicular line and midaxillary line.
Diaphragmatic eventration is a thinning of a portion or all of the diaphragm where muscle has been replaced by fibroelastic tissue. It is prudent that the clinician recognizes this condition so that appropriate evaluation and treatment can be instituted. The causes, diagnostic evaluation, and treatment of diaphragm eventration in adults will be ...
The diaphragm (dia: across, phragm: fence) is the musculotendinous boundary between the negative-pressure thoracic cavity and positive-pressure abdominal cavity. The diaphragm plays a significant role in respiratory mechanics, and injury to the diaphragm impairs ventilation and oxygen delivery. The normal mechanics of respiration are discussed ...
Ultrasound assessment of respiratory muscle. A: Transducer placement for assessment of parasternal intercostal thickening fraction.B: Inspiratory (yellow line 1) and expiratory (yellow line 2) intercoastal muscle thickness were measured using the M-mode.C: Transducer placement for assessment of diaphragmatic excursion.D: The amplitude of diaphragmatic muscle (yellow arrow) excursion between ...
matic excursion and thickness. A few studies have assessed diaphragmatic kinetics in children with diaphragmatic paresis and paralysis, but to our knowledge, no data about normal values in pediatrics are available. The aims of this study were to determine ref-erence values for diaphragmatic excursion and thickness, as evaluated by sonography in
The mean T 0 diaphragm excursion was slightly larger in NIV-responder patients (mean DE 1.35 ± 0.78 cm) compared to non-responders ... before NIV treatment may have a worse response to NIV than patients with a greater muscle functional reserve. In our study NIV responder patients demonstrated longer respiratory times at T 0 ...
The diaphragm is a unique skeletal muscle; it is active throughout the lifetime and, therefore, differs from locomotor muscles in the properties of muscle fibers and the mechanisms of blood supply control. ... Suzuki, J., Microvascular remodelling after endurance training with Co 2+ treatment in the rat diaphragm and hind-leg muscles, Jpn. J ...
Normal diaphragm excursion is between 3 and 5 cm. An abnormal sniff test is the paradoxic motion of a hemidiaphragm upward by greater than 2 cm which can indicate diaphragm paralysis (Fig. 3A, B ). The sniff test is positive in 90% of patients with unilateral phrenic nerve palsy.
The contrast-enhanced MDCT revealed a large, well-circumscribed lesion of the right hemidiaphragm deforming upper contour of the liver. A clear boundary between the lesion and the liver suggested former's diaphragmatic origin. PET-CT did not show any distant metastasis. Intraoperative revision revealed a tumor growing from the dome of the ...
Diaphragmatic excursion and lung function were analyzed by an independent t-test. To analyze the relationship between lung function and diaphragmatic excursion, Karl Pearson's correlation coefficient test was used. The level of significance was <0.05 (P < 0.05). Results. Forty-eight participants were included in the study. Out of those, 30 were ...
M.A. Chicherina's 6 research works with 2 citations and 39 reads, including: THE DIAPHRAGM EVENTRATION UNDER THE GUISE OF RECURRENT PARAESOPHAGEAL HIATAL HERNIA
The push into Kursk may force Russia into a decision to treat the border as a front rather than a dormant area, with implications for Moscow's deployment of personnel and materiel within Ukraine.
Treatment modalities include both pharmacological and non-pharmacological interventions. ... Diagnosis of diaphragmatic dysfunction includes ultrasound values of TF <20-29% and diaphragmatic excursion of less than 1 cm. Reduced, absent or paradoxical diaphragmatic motility supports the diagnosis of diaphragmatic palsy. Reduced Tdi-exp ...